- 1MARE – Marine and Environmental Sciences Centre, ESTM, Polytechnic Institute of Leiria, Peniche, Portugal
- 2Biology Department, Do*Mar Doctoral Programme on Marine Science, Technology and Management, University of Aveiro, Aveiro, Portugal
- 3Faculty of Sciences, BioISI - Biosystems and Integrative Sciences Institute, University of Lisboa, Lisbon, Portugal
- 4Faculty of Sciences and Technology, University of Coimbra, Coimbra, Portugal
- 5Center of Investigation in Environment, Genetics and Oncobiology, University of Coimbra, Coimbra, Portugal
- 6Center for Neuroscience and Cell Biology, University of Coimbra, Coimbra, Portugal
- 7Departament of Pharmacology, Faculty of Veterinary, University of Santiago de Compostela, Lugo, Spain
Marine environment has demonstrated to be an interesting source of compounds with uncommon and unique chemical features on which the molecular modeling and chemical synthesis of new drugs can be based with greater efficacy and specificity for the therapeutics. Cancer is a growing public health threat, and despite the advances in biomedical research and technology, there is an urgent need for the development of new anticancer drugs. In this field, it is estimated that more than 60% of commercially available anticancer drugs are natural biomimetic inspired. Among the marine organisms, algae have revealed to be one of the major sources of new compounds of marine origin, including those exhibiting antitumor and cytotoxic potential. These compounds demonstrated ability to mediate specific inhibitory activities on a number of key cellular processes, including apoptosis pathways, angiogenesis, migration and invasion, in both in vitro and in vivo models, revealing their potential to be used as anticancer drugs. This review will focus on the bioactive molecules from algae with antitumor potential, from their origin to their potential uses, with special emphasis to the alga Sphaerococcus coronopifolius as a producer of cytotoxic compounds.
Introduction
Natural products (NPs) have been used as therapeutic agents for the treatment of a wide spectrum of illnesses for thousands of years, playing an important role in meeting the basic needs of human populations. In 1985, World Health Organization estimated that ~65% of world population ensured their primary health care using predominately plant-derived traditional medicines, existing a less prevalence in developed countries (Cragg and Newman, 2013). Due their unusual chemical features, NPs have functioned as scaffolds for the development of new products with huge therapeutic and industrial potential. Moreover, these compounds present a greater efficiency and specificity with target sites since were originated in co-evolution with biological systems. These interesting compounds result from the interactions between organisms and their environment, which promote the production of diverse complex chemical compounds by the organisms to increase their survival and competitiveness (Mishra and Tiwari, 2011).
Comparing with terrestrial organisms, marine organisms do not have a distinguished history of use in traditional medicine. However, in the last 50 years, advances in new technologies and engineering such as scuba diving techniques, manned submersibles and remotely operated vehicles (ROVs) opened up the marine environment to scientific exploration (Cragg and Newman, 2013). The coexistence of several species in these habitats of limited extent increases their competitiveness and complexity. For example, sessile organisms such as algae, corals, sponges, and other invertebrates are in constant competition and many of them have evolved chemical means to defend themselves against predation or overgrowth of competing species or, conversely, to subdue motile prey species for ingestion. These chemical adaptations are generally defined as “secondary metabolites” and involve different classes of chemical compounds, which have evidenced great pharmacological potential (Simmons et al., 2005). Therefore, marine organisms have revealed to be an exceptional reservoir of NPs, some of them with different structural features from those of terrestrial sources. Despite considerable challenges, some marine compounds arrived in the market and are currently used in therapeutics, providing a useful roadmap for future translational efforts (for details, please see topic 4; Arizza, 2013). Among the different illnesses, cancer is a growing threat to public health, particularly in developed countries, and it is expected that their occurrence and associated deaths will increase in the next years (American Cancer Society, 2015). This problem is directly associated with the growth and aging of the population and the adoption of behaviors that contribute to increase cancer risk (American Cancer Society, 2015). Moreover, owing to the tumor cells resistance to drugs, significant toxicity, and undesirable side effects observed with synthetic drugs, there is an urgent need for new antitumor drugs development (Sawadogo et al., 2015; Torre et al., 2015). Given that cancer is a multifactorial and multi-targeting disease that cannot be prevented by mono-targeted therapies, many researchers have focused their efforts toward NPs, especially those from marine environments, to identify novel anticancer compounds. Today, it is estimated that more than 60% of anticancer drugs presently in the market are of natural origin (Cragg and Newman, 2009). In addition, there are several natural compounds originated or derived from marine life presently undergoing clinical trials with oncological indications (AndisInsight, 2018; Calado et al., 2018; EMA, 2018a,b; FDA, 2018a,b; Mayer, 2018).
This review contains six topics condensing the importance of marine resources as source of antitumor compounds, summarizing the most important works accessing the potential of algae as source of marine drugs for cancer therapeutics.
Cancer Biology—General Overview
Cancer is one of the major human health problems worldwide, with high social and economic impacts. There is evidence of this disease in antiquity, dating back to the times of the Pharaohs in ancient Egypt and the classical world (Nobili et al., 2009). Currently, worldwide, cancer is responsible by one in each seven deaths causing more deaths than AIDS, tuberculosis and malaria combined (American Cancer Society, 2015). Only surpassed by cardiovascular diseases, cancer is the second leading cause of death in high-income countries while being the third leading cause of death in low- and middle-income countries following cardiovascular, infectious and parasitic diseases (American Cancer Society, 2015). By 2030, it is estimated that the incidence of this illness grows to over 21.7 million new cases and 13 million deaths (American Cancer Society, 2015). Beyond the social impact, cancer is also associated with high financial costs at the individual and social level for both the person with cancer and for society as a whole. For example, in 2011 in the US the direct medical costs (total of all health care expenditures) associated with cancer was estimated in $88.7 billion, which are related with hospital outpatient or office-based provider visits, patient hospital stays and medical prescriptions (American Cancer Society, 2015).
However, what we simply call “human cancer” comprises, in fact, more than 100 different diseases that result from the continuous uncontrolled proliferation of cancer cells (Urbano et al., 2011), which have the capability to invade organs and normal tissues, as well as metastasizing through the body. These cells do not respond properly to the signals that regulate their normal behavior (Schulz, 2007; Cooper and Hausman, 2013). In line with this view, Hanahan and Weinberg (2000) published a review article that combined information about cancer biology and defined six hallmarks (sustaining proliferative signaling, resisting cell death, inducing angiogenesis, enabling replicative immortality, activating invasion and metastasis and evading growth suppressors) that all cancer cells have and that are responsible for their malignant properties. Subsequently, an upgrade of this list was done adding two new hallmarks, deregulating cellular energetics and evading immune destruction. Nevertheless, the occurrence of these hallmarks is directly associated with the genome instability, which is responsible by genetic diversity that stimulates their acquisition, and inflammation and promotes multiple hallmark functions (Hanahan and Weinberg, 2011). Recently, case–control metagenomics studies suggest that dysbiosis in the commensal microbiota is also associated with various cancer types adding microbiome as an additional hallmark (Rajagopala et al., 2017). The complexity of tumors represents a great challenge for therapeutic approaches, as experimental evidence exists that each core hallmark capability is regulated by partially redundant signaling pathways (Hanahan and Weinberg, 2011). Consequently, targeted therapy mediated by drugs that only act on one key pathway in a tumor may not be enough to “switch off” a hallmark capability completely. As a result some cancer cells can survive maintaining a basal function awaiting an adaptation of their progeny to the selective pressure imposed by the drug. This adaption can be accomplished by genetic changes, epigenetic reprogramming, or remodeling of the stromal microenvironment. All of these processes can contribute for restitution of the functional capability, allowing renewed tumor growth and consequently clinical relapse (Hanahan and Weinberg, 2011). Nevertheless, the drug resistance of tumor cell lines can also be mediated by other mechanisms, such as drug efflux, suppression of drug activity, changes in cellular targets, enhanced DNA repair, inability to induce cell death and the epithelial-mesenchymal transition (Housman et al., 2014). Among all the treatments currently used in cancer (surgery, radiotherapy, hormonal treatment and immunotherapy, adjunct therapy, and chemotherapy), chemotherapy continues to play an extremely important role. However, its effectiveness is limited in some cases by the existence of drug resistance, making it necessary to define optimal combinations for therapeutic strategies that ensure an efficient elimination of the tumor. Moreover, in the last decades, with the continuous growth of cancer cases and concerns over toxicity, tumor cell resistance, the development of secondary cancers and the unwanted side effects observed with synthetic drugs, there has been an increased interest in exploiting NPs for cancer treatment (Newman and Cragg, 2012; Sawadogo et al., 2015).
Role of the Marine Chemical Ecology in the Production of Bioactive Metabolites on Algae
The oceans represent a vast area of the planet and play a fundamental role in its dynamic. Their physics, chemistry and biology are key elements in the functioning of the earth system, providing an interconnection between the different natural systems (terrestrial, freshwater, estuarine, coastal, and oceanic) and a range of valuable ecosystem services (Atkins et al., 2011; Halpern et al., 2012; Botana and Alfonso, 2015). Their essential role is further noted by the significant fraction of the Earth's biodiversity that oceans harbor (Brahmachari, 2015). According to the 33 animal phyla listed by Margulis and Chapman (2009), 32 of them are represented in aquatic environments, with 15 exclusively marine, 17 found in marine and non-marine environments (with 5 of these having more than 95% of their species only in marine environments), and only one exclusively non-marine (Onychophora). A recent study predicted the existence of ~8.9 million eukaryotic species, of which ~2.2 million are marine organisms, suggesting that around 86% of the species on the earth, and 91% in the ocean, have not yet been described (Mora et al., 2011; Cragg and Newman, 2013; Berkov et al., 2014). The existence of a huge diversity of life forms in the oceans is associated with the very exigent, competitive and aggressive surrounding that promotes specific and complex interactions, both inter-species and intra-species. During the evolutionary period, many species share a common environment establishing well-balanced associations between them. Inside of these communities several organisms survive and live in close association with other species, both macro (e.g., algae, sponges, and ascidians) and micro (e.g., bacteria, fungi, and actinomycetes) in order to ensure their survival (Da Cruz et al., 2012; Graça et al., 2013; Horta et al., 2014; Smith et al., 2018). Many of these complex interactions are mediated by chemical signals, which play a crucial role at the organizational level in the marine environment (Hay, 2009). These chemical cues constituting much of the language of sea life and are the utmost importance for several marine species which have not some senses such as vision and hearing; nevertheless even species that see and hear rely on chemical cues (Botana and Alfonso, 2015). Interactions mediated by chemical signals play a crucial and decisive role in ecological processes. These signals influence population structure, community organization and ecosystem function. In addition they are involved in the definition of escape strategies, commensal associations, partners and habitats, competitive interactions, feeding choices and energy and nutrients transfer within and among ecosystems (Hay, 2009).
Among marine organisms, algae are a clear example in which chemical signals play a fundamental role in ecological processes. These signs are involved in the growth and survival in extremely exigent conditions, giving them competitive advantages relative to other marine organisms including against predators and competitors. One such piece of evidence has recently been observed in coral reefs, which are in dramatic global decline, with algae commonly replacing them. Several studies have observed that algae damage corals directly or colonize opportunistically, suppressing coral recruitment through the production of specific chemical cues repulsing the recruits (Rasher and Hay, 2010, 2014; Dixson et al., 2014). Recently, Rasher et al. (2011) identified four compounds (two loliolide derivatives and two acetylated diterpenes) from two algae as potent allelochemicals which directly damage corals. Marine organisms sometimes face the dilemma of how to allocate the limited resources available, having to strategically adapt. Usually these decisions may have consequences on their growth, reproduction, or ability to counteract biological (e.g., predators, maintenance of unfouled surfaces, paralyzing their prey, etc.) and/or physical stress (e.g., UV light, temperature, nutrient availability, high pressure, salinity, oxygen content, etc.) (Winter et al., 2010; Botana and Alfonso, 2015). The production of these types of compounds has also been revealed to be an important weapon for the successful invasion of non-indigenous species into new ranges. For instance, the invasive red alga Bonnemaisonia hamifera has become one of the most abundant species in Scandinavian waters. According to Svensson et al. (2013) the high capacity of this alga to colonize these waters seems to be linked with the presence of a specific chemical compound (1,1,3,3-tetrabromo-2-heptanone). The production of this metabolite inhibit the settlement of propagules on its thallus and on surrounding surfaces, achieving a competitive advantage over native algae (Svensson et al., 2013). Chemical cues also play an important role in the symbiotic interactions established between algae and microorganisms. The cross-kingdom interactions between them are not restricted to the exchange of macronutrients, including vitamins and nutrients but also include the use of infochemicals with different functions, establishing a tight relationship and enabling them to interact as a unified functional entity (Egan et al., 2013; Wichard, 2015). For instance, associated microorganisms are responsible to produce compounds of utmost importance which mediate essential ecological functions in the development and growth of algae species including quorum sensing signaling molecules, compounds with biological activities, substances that promote the growth and other effective molecules compounds (Singh and Reddy, 2014). Some of these compounds, such as bacterial morphogenetic compounds, dimethylsulfoniopropionate (DMSP), the amino acids proline and alanine, halogenated furanones and fucoxanthin, play important roles in the ecological function, interfering with the surface fouling of others organisms as well as with the vital functions performance of the algae. These compounds prevent the attachment of certain bacteria (e.g., Cytophaga sp.) and support the fixation of others (e.g., Rheinheimera baltica), controlling the community composition and abundance of the algae-associated bacteria (Saha et al., 2011, 2012; Spoerner et al., 2012; Egan et al., 2013). Marine organism interactions promote the production of a high diversity of marine NPs with quite specific and potent activities, representing an enormous source of new compounds with potential for biotechnology applications providing economic and human benefits.
Marine Natural Products as a Source of New Drugs and Current Clinical Pipeline
Along of the evolution, marine organisms developed exceptional metabolic capacities through the production of compounds with quite specific and potent activities (Murray et al., 2013; Martins et al., 2014). These compounds, often defined as secondary metabolites, are generally limited to a particular taxonomic family, genus, species or even organism, characterized by their wide heterogeneity, and often constitutes a very small fraction of the total biomass of the organism (Ianora et al., 2006; Avila et al., 2008; Martins et al., 2014). Predominantly, their production occurs in sessile or slow-moving organisms (e.g., algae, sponges, cnidarians, tunicates and bryozoans) that, without effective escape mechanisms or structural protection, ensure their protection through chemical defense (Noyer et al., 2011; Botana and Alfonso, 2015). Nevertheless, many organisms have the capacity to sequester secondary metabolites from their diet and then derivatize them to more or less toxic forms can be these used for functions different from their roles in the original producer (Ianora et al., 2006; Kicklighter et al., 2011; Botana and Alfonso, 2015; Gotsbacher and Karuso, 2015). Moreover, since natural compounds released into the water are rapidly diluted, they need to be highly potent to retain their efficacy (Haefner, 2003). For these reasons, it is widely accepted that a huge number of NPs and novel chemical entities that exist in the oceans could be useful for providing sustainable economic and human benefits.
To date, different types of secondary metabolites (e.g., terpenoids, alkaloids, polyketides, peptides, shikimic acid derivatives, sugars, steroids, and a large mixture of biogenesis metabolites) were isolated from marine organisms and found to exhibit many biological activities (antimicrobial, antitumor, antidiabetic, anticoagulant, antioxidant, anti-inflammatory, antiviral, antimalarial, antitubercular, anti-aging antifouling, and antiprotozoal) with huge industrial and therapeutic potential (Blunt et al., 2013, 2014; Mayer et al., 2013, 2017; Agrawal et al., 2018). Marine NPs have exhibited rare and unique chemical structures, upon which the molecular modeling and chemical synthesis of new drugs can be based on (Dias et al., 2012; Botana and Alfonso, 2015). Since this kind of compounds are originated from nature present several advantages compared with synthetic compounds such as a chemical diversity, biochemical specificity, binding efficiency, and affinity to interact with biological systems, making them interesting structures for development of new drugs (Martins et al., 2014).
The marine biodiscovery and vision of marine-derived drugs on the market had their beginning in the early 1950s with Bergmann, who isolated and identified two nucleosides, spongouridine and spongothymidine, from the Caribbean sponge Cryptotethya crypta (previously known as Tethya crypta). These discoveries led researchers to synthesize analogs, Ara-A (Vidarabine®, Vidarabin Thilo®) and Ara-C (Cytarabine, Alexan®, Udicil®), the first marine derived compounds that have reached the market as antiviral and antitumor drugs, respectively, (Newman et al., 2009; Botana and Alfonso, 2015). Over the last 50 years was reported the isolation of more than 30,000 new compounds of marine origin (Figure 1) and the approval of more than 300 patents (Blunt et al., 2015; Botana and Alfonso, 2015).
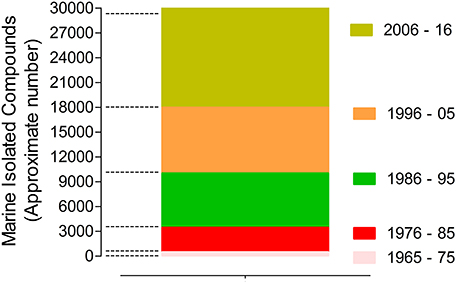
Figure 1. Marine compounds isolated in the last 50 years (approximate number/10 years) (Faulkner, 1984, 1986, 1987, 1988, 1990, 1991, 1992, 1993, 1994, 1995, 1996, 1997, 1998, 1999, 2000, 2001, 2002; Blunt et al., 2003, 2004, 2005, 2006, 2007, 2008, 2009, 2010, 2011, 2012, 2013, 2014, 2015, 2016, 2017, 2018).
Nevertheless, despite of the remarkable potential of marine NPs as source of new drugs their role have undergone several changes, having had an evident decline in the pharmaceutical R&D activities by the mid-1990s. After that decline, the larger research and development effort in the exploration of this niche was essentially assured by enterprising academics, mainly partnered with industry. In the last decade, this area seems to have benefited from a renaissance, since the number of new isolated marine compounds has increased when compared with the previous similar period (Molinski et al., 2009; Botana and Alfonso, 2015). This rebirth is directly associated with recent technological advances in analytical technology, spectroscopy, and high-throughput screening. Advances in “omics” techniques (genomics, metagenomics, proteomics), combinatorial biosynthesis, synthetic biology, selection methods, expression systems, and bioinformatics have contributed as powerful tools to discover new chemical entities with pharmaceutical potential (Molinski et al., 2009; Bucar et al., 2013).
Over the last 30 years, great efforts have been made, showing productive and promising results, since it has been defined the major tendencies in secondary metabolism of several classes of marine organisms. Only in the last 20 years, more than 18,000 new marine compounds were described and six (Figure 2) out of the nine marine-derived drugs currently used in clinical therapy were approved, as well as one over-the-counter drug (OTC). Cytarabine (Cytosar-U®), Vidarabine (Vira-A®) (US discontinued), Ziconotide (Prialt®), Brentuximab Vedotin (Adcetris®), Eribulin Mesylate (Halaven®), Omega-3-acid ethyl esters (Lovaza®), Trabectedin (Yondelis®), Fludarabine Phosphate (Fludara®), and Nelarabine (Arranon®) were approved by the Food and Drug Administration (FDA) in the US Pharmacopeia and/ or by the European Agency for the Evaluation of Medicinal Products (EMA). Iota-carrageenan (Carragelose®), one OTC, was approved by EMA. However, it is expected that the number of newly approved drugs from marine origin will continue to increase, since 28 marine or marine-derived drugs are currently in clinical trials (six marine molecules—phase III; fourteen marine molecules—phase II; eight marine molecules—phase I) (Figure 2) (AndisInsight, 2018; Calado et al., 2018; EMA, 2018a,b; FDA, 2018a,b; Mayer, 2018).
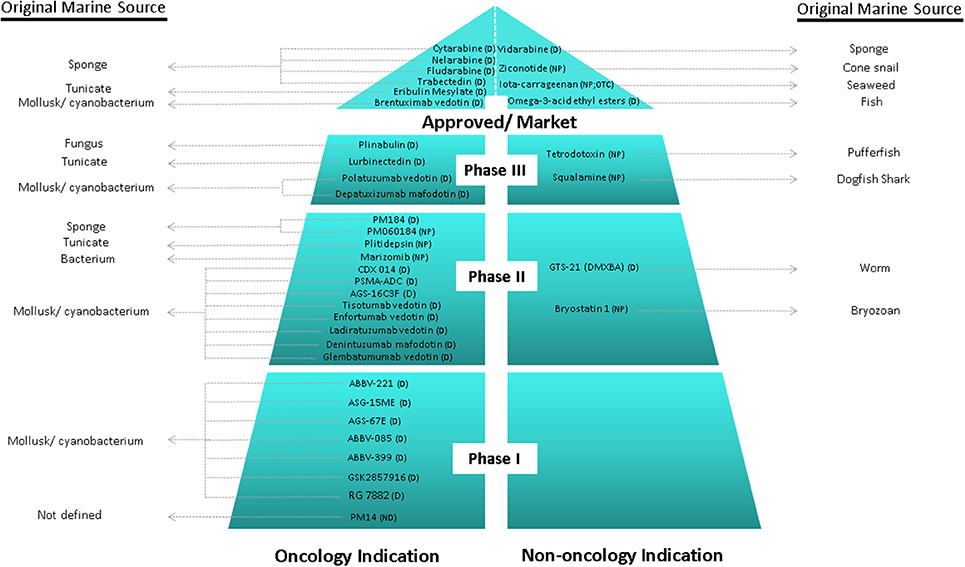
Figure 2. Current clinical pipeline of marine-derived drugs and their original marine source. NP, Natural product; D, Derivate; OTC, Over-the-counter (AndisInsight, 2018; Calado et al., 2018; EMA, 2018a,b; FDA, 2018a,b; Mayer, 2018).
Considering all the marine drugs available in the market, it is particularly interesting to see that six are used in cancer therapies (Figure 3), and the majority of the compounds that are in clinical trials are also for application in cancer therapy, which reveals the great potential of marine compounds as anticancer drugs (Figure 2).
Although not associated with cancer therapeutics, iota-carrageenan (Carragelose®) is the first product developed from algae in the market. It is a type of carrageenan, isolated from a red edible algae belonging a family of linear sulfated polysaccharides. Carragelose® has ability to block viral attachment to the host cells being effective against a broad spectrum of respiratory viruses (Ludwig et al., 2013; Calado et al., 2018).
Antitumor Potential of Marine Algae-Derived Compounds
Among marine organisms, algae are one of the most important resources of the ocean, economically and ecologically (Kim, 2014). Their inclusion in the Asian diet has been associated with health benefits, where there has been observed a lower incidence of chronic diseases, such as hyperlipidaemia, coronary heart disease, diabetes and cancer, according to epidemiological studies comparing Japanese and Western diets (Brown et al., 2014; Bouga and Combet, 2015). Algae are valuable sources of protein, fiber, vitamins, polyunsaturated fatty acids, and macro-, and trace elements. More recently, they have also revealed to be an interesting source of useful bioactive components such as antioxidants, phycocolloids, proteins, vitamins, minerals, carotenoids, soluble dietary fibers, polyunsaturated fatty acids, phycobilins, polysaccharides, sterols, tocopherols, terpenes, and phycocyanins. These compounds demonstrated to possess nutritional and functional value apart from their potential use as therapeutic agents in biomedical area (Chandini et al., 2008; Lordan et al., 2011; Mohamed et al., 2012; Alves et al., 2016b). Due to their unique structures and biochemical characteristics, the multifunctional properties of algae should be exploited in their fullness. In addition, the idea that algae are a promising prolific source of structurally unique NPs with biomedical potential is even more supported by the view that the number of algae species identified around the world is more than 30,000 (Plouguerné et al., 2014). Moreover, algae have been revealed a major source of new compounds of marine origin, after sponges, microorganisms and phytoplankton (Figure 4).
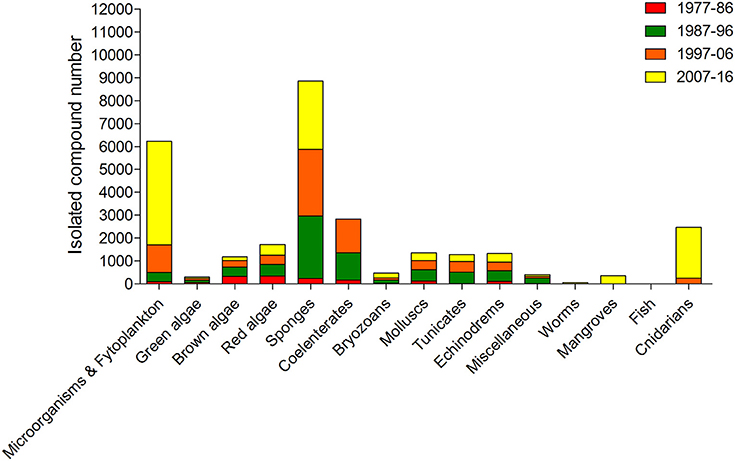
Figure 4. Approximate numbers of new compounds isolated from different marine organism sources between 1977 and 2016 (Faulkner, 1984, 1986, 1987, 1988, 1990, 1991, 1992, 1993, 1994, 1995, 1996, 1997, 1998, 1999, 2000, 2001, 2002; Blunt et al., 2003, 2004, 2005, 2006, 2007, 2008, 2009, 2010, 2011, 2012, 2013, 2014, 2015, 2016, 2017, 2018).
Many of these algae-derived compounds have proven therapeutic properties associated with numerous health-promoting effects, including anti-obesity (Maeda et al., 2005; Kim et al., 2018), antidiabetic (Mayer et al., 2013), antihypertensive (Sivagnanam et al., 2015), antihyperlipidaemia (Sathivel et al., 2008), antioxidant (Magalhaes et al., 2011; Pinteus et al., 2017), anticoagulant (Magalhaes et al., 2011), anti-inflammatory (De Souza et al., 2009), immunomodulatory (Pérez-Recalde et al., 2014), anti-estrogenic (Skibola, 2004), thyroid-stimulating (Teas et al., 2007), neuroprotective (Pangestuti and Kim, 2011), anti-osteoarthritic (Moon et al., 2018), anti-osteoporosis (Deng et al., 2018), antiviral (Aguilar-Briseño et al., 2015), antimicrobial (Pinteus et al., 2015; Rodrigues et al., 2015), and antitumor (Moussavou et al., 2014; Rodrigues et al., 2015; Alves et al., 2016a). Among the well-documented bioactive compounds are brominated phenols, polysaccharides, and carotenoids, but especially a large diversity of terpenoids, several of them being halogenated compounds (Gribble, 2015; Rodrigues et al., 2015). Although many works have attempted to identify marine-derived compounds, detailed chemical characterization and identification of bioactive components are still largely lacking (Santos et al., 2015).
Along the last five decades, it is estimated that more than 3,000 NPs have been discovered from algae (Leal et al., 2013), and among all of the biological activities observed, the antitumor activity is one of the most promising. Despite several studies have shown the high cytotoxic potential of the compounds isolated from algae on different tumor cell lines, there are few studies that have characterized the intracellular signaling pathways involved in the process (Table 1). Currently, according to the National Cancer Institute (USA), different targeted therapies have been approved for use in cancer treatment, including hormonal therapies, inhibitors of signal transduction and angiogenesis, modulators of gene expression, inducers of apoptosis, immunotherapies and toxin delivery molecules.
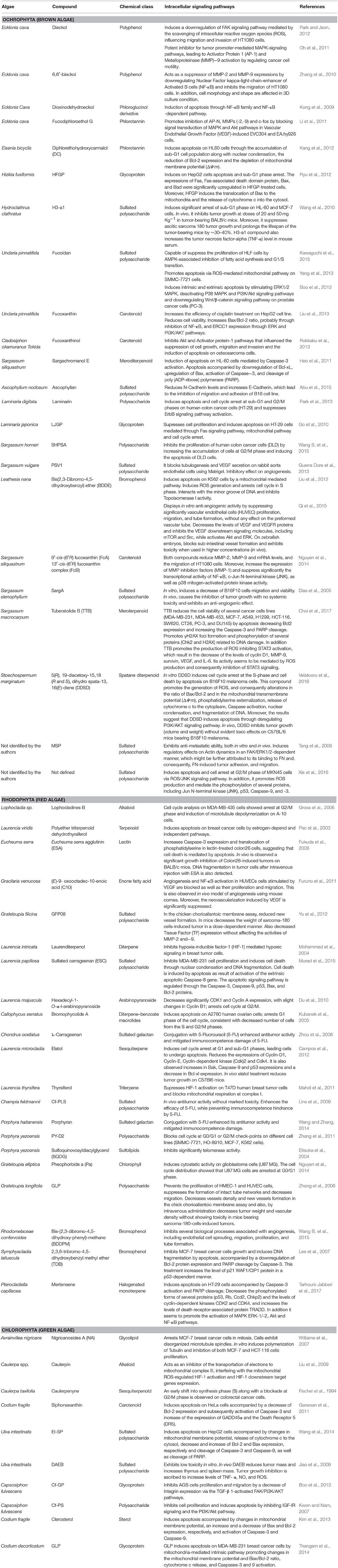
Table 1. Marine compounds isolated from algae with antitumor and cytotoxic activities and intracellular signaling pathways involved.
In vitro Antitumor Activities of Algae-Derived Compounds and Intracellular Signaling Pathways Activated
Analyzing the compounds isolated from algae with antitumor activity (Table 1) is possible to see that several of them mediate their activities in some of these target therapies mentioned previously. For example, dioxinodehydroeckol (Kong et al., 2009), sargachromanol E (Heo et al., 2011), EI-SP (Wang et al., 2014), siphonaxanthin (Ganesan et al., 2011), sulfated carrageenan (Murad et al., 2015), TDB (Lee et al., 2007), GLP (Thangam et al., 2014), mertensene (Tarhouni-Jabberi et al., 2017), TTB (Choi et al., 2017), DDSD (Velatooru et al., 2016), and clerosterol (Kim et al., 2013) induced apoptosis in different cell lines by similar intracellular signaling pathways, regulated by Caspase (−3, −9 or both) activation, downregulation of Bcl-xL or Bcl-2, upregulation of Bax and cleavage of PARP. Moreover, some of these compounds, such as EI-SP and clerosterol, also caused the loss of the mitochondrial membrane potential. The treatment of colon26 cells with Eucheuma serra agglutinin (Fukuda et al., 2006) also promoted an increase of Caspase-3 expression and translocation of phosphatidylserine in lectin-treated cells, suggesting that cell death was mediated by apoptosis. On the other hand, algae-derived compounds such as lophocladines B (Gross et al., 2006), hexadecyl-1- O-α-l-arabinopyranoside (Du et al., 2010) and caulerpenyne (Fischel et al., 1994) affected the intracellular signaling pathways linked with regulation of the cell cycle. Lophocladines B showed a marked reduction of MDA-MB-435 cells at the G1 and S phases, with an accumulation of cells at G2/M, indicating a G2/M cell cycle arrest. This compound also induced microtubule depolymerization on A-10 cells. Du et al. (2010) isolated from the alga Laurencia majuscula a new arabinopyranoside compound designed as hexadecyl-1- O-α-l-arabinopyranoside, which exhibited significant antitumor activity in different cancer cell lines. The active compound arrested cell lines at G2/M phase of the cell cycle by decreasing the expression of CDK1 and Cyclin A proteins, which are critical for the G2/M-phase transition. Caulerpenyne induced cell cycle arrest in colorectal cancer cells that exhibited an early shift into the S phase followed by a blockade at the G2/M phase (Fischel et al., 1994).
Nevertheless, most of the intracellular signaling pathways activated by these compounds are simultaneously linked with the regulation of cell cycle and apoptosis. Park et al. (2013) demonstrated that laminarin, extracted from brown alga Laminaria digita, induced apoptosis on HT-29 colon cancer cells and increased the percentage of cells in the sub-G1 and G2/M phases. The observed decrease in cellular proliferation was found to be dependent on ErbB, followed by subsequent activation of c-Jun N-terminal kinase. In the same way, Liu et al. (2012) verified that a bromophenol compound, bis(2,3-dibromo-4,5- dihydroxybenzyl) ether, induced apoptosis on K562 cells by a mitochondria-mediated pathway, as well as the arrest of the cell cycle at the S phase. Additionally, this compound interacted with the minor groove of DNA and inhibited Topoisomerase I activity. On A2780 human ovarian cells, bromophycolide A induced the arrest at the G1 phase of the cell cycle and a consequent and consistent loss of cells from the S and G2/M phases, while simultaneously induced apoptosis (Kubanek et al., 2005). Similar effects were induced by elatol, a compound isolated from the alga Laurencia microcladia, which induced cell cycle arrest in the G1 and sub-G1 phases, leading the cells to undergo apoptosis. It influenced the expression of several proteins (cyclins, Bax, Bcl-xl, caspases, p53) that play important roles in these biological processes (Campos et al., 2012). Diphlorethohydroxycarmalol, isolated by Kang et al. (2012), induced apoptosis by the accumulation of the sub-G1 cell population and nuclear condensation, depletion of mitochondrial membrane potential (ΔΨm) and regulation of the expression of the pro-survival and pro-apoptotic Bcl-2 family members. HFGP (Ryu et al., 2012) and LJGP (Go et al., 2010) glycoproteins induced apoptosis on HepG2 and HT-29 cells, which was mediated by Fas signaling and mitochondrial pathway, and cell cycle arrest. On the other hand, Cf-PS polysaccharide (Kwon and Nam, 2007) inhibited the cell proliferation and induced apoptosis by inhibiting IGF-IR signaling and the PI3K/Akt pathway, which are involved in the regulation of cell growth, proliferation, differentiation, motility, survival, metabolism and protein synthesis (Chen et al., 2014).
Other examples such as dieckol (Oh et al., 2011; Park and Jeon, 2012), 6,6′-bieckol (Zhang et al., 2010), ascophyllan (Abu et al., 2015), 9′-cis-(6′R) fucoxanthin (FcA) (Nguyen et al., 2014), fucoxanthinol (Rokkaku et al., 2013), and 13′-cis-(6′R) fucoxanthin complex (FcB) (Nguyen et al., 2014), SargA (Dias et al., 2005), MSP (Tang et al., 2006), and Cf-GP (Boo et al., 2013) showed the capacity to inhibit the motility, migration, adhesion or invasion on different in vitro and in vivo models using distinct intracellular signaling pathways, as described in Table 1.
Currently, one of the targets of cancer treatment, especially in solid tumors, is angiogenesis, which is responsible for the formation of new blood vessels and is a requirement for the sustained growth and proliferation of solid tumors. Accordingly, the search for inhibitors of this process has become a leading line of investigation in anticancer research, with the consequent release of several drugs on the market that have clearly improved outcomes in patients with different tumor types and metastatic disease (Marín-Ramos et al., 2015). The compounds PSV1 (Guerra Dore et al., 2013), SargA (Dias et al., 2005), BDDE (Qi et al., 2015), GFP08 (Yu et al., 2012), GLP (Zhang et al., 2006), Fucodiphloroethol G (Li et al., 2011), C10 (Furuno et al., 2011), and BDDPM (Wang B. et al., 2015) also demonstrated interesting anti-angiogenic activities. For example, the sulfated polysaccharide, PSV1, inhibited tubulogenesis in RAEC cells in Matrigel and VEGF secretion (Guerra Dore et al., 2013); SargA induced a marked dose-dependent inhibition of capillary networks development (Dias et al., 2005). As to fucodiphloroethol G, this compound inhibit angiogenesis on ECV-304 and EA.hy926 cells when induced with VEGF, as well as the transcriptional factor c-fos and its targets AP-N, MMP-2, by MAPK, and Akt signaling pathways inhibition (Li et al., 2011). However, one of the most interesting and promising compounds is BDDPM, reported to inhibit various biological processes associated with angiogenesis, including endothelial cell sprouting, migration, proliferation, and tube formation (Wang B. et al., 2015). Kinase assays revealed that BDDPM is a potent selective but multi-target receptor tyrosine kinase (RTKs) inhibitor (VEGFR, PDGFR, FGFR, and EGFR). However, other compounds have not only evidenced in vitro activity but also in vivo as described in the section Preclinical and Clinical Evidence of Antitumor Activities of Algae-Derived Compounds of the present review.
Compounds such as laurenditerpenol (Mohammed et al., 2004), caulerpin (Liu et al., 2009), thyrsiferol (Mahdi et al., 2011), phlorofucofuroeckol-A (Lee et al., 2012), SQDG (Eitsuka et al., 2004), and DAEB (Jiao et al., 2009) showed antitumor activity by activating other intracellular signaling pathways. For example, laurenditerpenol, thyrsiferol, and caulerpin showed the capacity to inhibit the transcription factor HIF-1 by blocking the induction of the oxygen-regulated HIF-1α protein, which promotes tumor cell adaptation and survival under hypoxic conditions (Ke and Costa, 2006). Lee et al. (2012) observed that phlorofucofuroeckol-A, a compound isolated from the edible brown alga Eisenia bicyclis, is a potent inhibitor of the aldo-keto reductase family 1 B10 (AKR1B10), a member of the NADPH-dependent aldo-keto reductase (AKR) superfamily, considered to be a potential cancer therapeutic target. In the same way, the compound SQDG showed a marked inhibition of the telomerase activity, which is an enzyme that drives the uncontrolled division and replication of cancer cells (Eitsuka et al., 2004).
Some of the compounds isolated from algae can also be used as co-adjuvants to improve the efficiency of the drugs currently used as therapeutics. For instance, the pre-treatment of HepG2 cells with fucoxanthin allowed to improve the therapeutic effect of cisplatin (Yang et al., 2013). According to Liu et al. (2013), these effects were associated with NFκB expression inhibition and an increase in the Bax/Bcl-2 mRNA ratios regulated by NFκB. Moreover, the decrease of the DNA repair systems regulated by ERK, p38, and PI3K/AKT seems also be associated with these effects. Additionally, the conjugation of λ-carrageenan (Zhou et al., 2006), Cf-PLS (Lins et al., 2009), and porphyran (Wang and Zhang, 2014) compounds with 5-FU drug enhanced its antitumor activity. According to previous studies, the conjugation of these compounds with 5-FU increased the antitumor activities of the drug and mitigated the immunocompetence damage induced by 5-FU (Zhou et al., 2006; Lins et al., 2009; Wang and Zhang, 2014).
Many of the studies discussed above have identified compounds, such as polysaccharides, polyphenols, carotenoids, alkaloids, terpenes and others, that mediate specific inhibitory activity on a number of key cellular processes, including apoptosis pathways, angiogenesis, migration and invasion processes, in different in vitro models revealing their potential use as anticancer drugs (Figure 5).
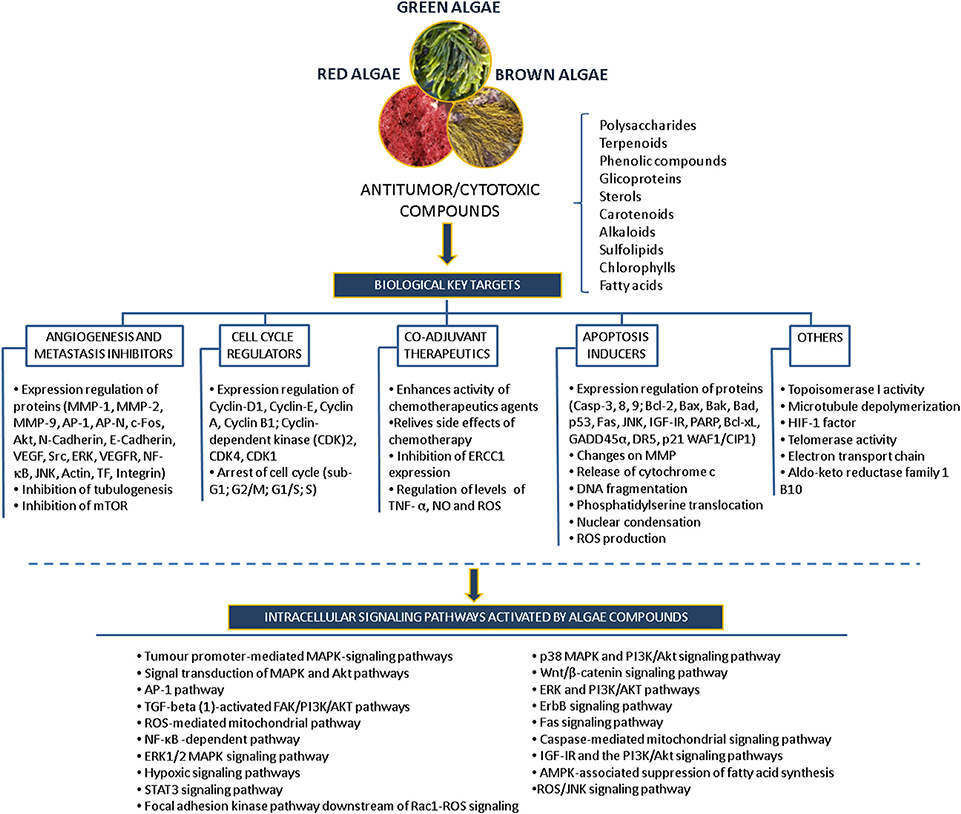
Figure 5. Overview of the antitumor/cytotoxic compounds isolated from algae, biological targets and intracellular signaling pathways activated.
Preclinical and Clinical Evidence of Antitumor Activities of Algae-Derived Compounds
Despite the antitumor activities of algae-derived compounds have majorly been described on in vitro human tumor models, there are several studies in preclinical and clinical trials demonstrating the potential of these compounds as antitumor and/or co-adjuvants drugs with capacity to act in distinct intracellular signaling pathways.
Among the chemical structures derived from algae, the highest number of in vivo studies were conducted with sulfated polysaccharides, revealing their potential to be used in antitumor therapies. For instance, the sulfated polysaccharides H3-a1 (Wang et al., 2010) and DAEB (Jiao et al., 2009) revealed antitumor activity by immune system enhancement, through the increase of the tumor necrosis factor-alpha (TNF-α) and ROS levels and by the activation of peritoneal macrophages leading to the secretion of TNF-α and NO. In addition, when administrated in tumor-bearing BALB/c mice at doses of 20 and 50 mg/Kg, the sulfated polysaccharide H3-a1 exhibited capacity to suppress the ascitic sarcoma 180 tumor growth, increasing the lifespan of the tumor-bearing mice in ~30–40%. On the other hand, the treatment with the sulfated polysaccharide DAEB reduced the thymus and spleen tumor growth in mice promoting the increase of immune organs weight.
Due to increasing evidences of the antitumor potential of algae derived sulfated polysaccharides, several studies have been conducted to deepen their antitumor potential, including studies addressing the possibility to be used as co-adjuvants drugs, to increase treatments efficiency and also as an attempt to reduce undesirable side-effects. As an example, the sulfated polyssacharide Cf-PLS extracted from the red alga C. feldmannii exhibited capacity to reduce the tumor growth on mice transplanted with sarcoma 180 tumor cells and to increase the leukocytes number in the peritoneal cavity and neutrophil migration. In addition, its co-administration with the chemotherapeutic agent 5-FU on tumor-bearing animals allowed to significantly increase the tumor growth inhibition, comparing with single administration, also preventing the immunocompetence hindered by 5-FU (Lins et al., 2009).
As described above, one of the targets for cancer treatment is angiogenesis. In these studies, several compounds have exhibited promising activities on in vivo models. The treatment of mice bearing sarcoma-180 cells with GLP polysaccharide promoted the reduction of tumor weight around 52% and the vascular density of the tumor (Zhang et al., 2006). In the same model, the GFP08 compound decreased the tumor weight by 68.9% and inhibited the formation of new vessels in the chicken chorioallantoic membrane assay (ex vivo) (Yu et al., 2012). By other side, the bromophenol BDDE also exhibited anti-angiogenesis properties by inhibiting sub-intestinal vessel formation in zebrafish embryos in vivo (Qi et al., 2015). Similarly to the bromophenol BDDE, the compound C10 inhibited markedly the neovascularization induced by VEGF in the mouse cornea (Furuno et al., 2011). On the other hand, the compound SargA inhibited the vascularization of Gelfoam skin implants and exhibited antitumor properties on melanoma cells with doses of 1.5 and 150 μg/animal without inducing deaths or body weight loss (Dias et al., 2005).
The MSP sulfated polysaccharide mediated a potent inhibitory effect on the metastasis of Lewis lung carcinoma reducing significantly the number of pulmonary metastatic colonies. The reduction of colony-formation rate was between 70 and 93.6%. Moreover, compared with cisplatin treatment, this compound promoted a significant amelioration of alveolar structures without inducing weight loss (Tang et al., 2006). DDSD (4, 10, and 15 mg/Kg) and Elatol [(oral (3, 10, 30 mg/Kg) or intraperitoneal (1, 3, 10 mg/Kg)] demonstrated significantly reduction of the tumor growth without evident toxic effects (Campos et al., 2012; Velatooru et al., 2016). The lectin ESA extracted from red alga Eucheuma serra, injected in the tail vein of BALB/c mice with colon26 cells, promoted a significant delay of the tumors growth inducing the cells death by apoptosis as observed in vitro. Additionally the treatment with ESA compound did not promoted weight loss or animal death (Fukuda et al., 2006). These studies become more relevant since the antitumor activities mediated by many of these compounds are not linked with toxic effects.
Other of the compounds widely studied on in vivo models, including clinical trials, is the sulfated polyssacharide fucoidan. This compound exhibits multi-targets acting in different signaling pathways, including the activation of the intrinsic and extrinsic pathways of apoptosis, suppression of angiogenesis, increase immune response, and mobilization of haematopoietic progenitor cells (Kwak, 2014; Moghadamtousi et al., 2014). The co-administration of low-molecular –weight fucoidan with standard drugs in patients with metastatic colorectal cancer improved the disease control rate suggesting its potential application as additional therapy (Tsai et al., 2017). In addition when administrated in conjugation with the standard hormonal drugs letrozole and tamoxifen, in patients with breast cancer, revealed to be well tolerated and without influence in the steady-state plasma concentrations of these drugs (Tocaciu et al., 2018). Despite de great potential demonstrated by fucoidans on clinical trials, to be used as suplementary therapy, its use as anticancer drugs is being studied, since fucoidan preparations obtained from different sources have induced different anticancer activities in vivo. These differential responses seem to be associated with their different structural properties. Therefore, it will be important to determine the structural characteristics of fucoidan responsible for the verified in vivo antitumor activities to ensure its potential use as therapeutic agent (Kwak, 2014).
The marine-derived cyclic depsipeptide kahalalide F was the first compound found in algae that achieved the phase II of clinical trials (Murphy et al., 2014). Kahalalide F is a potent cytotoxic compound produced by the green alga Bryopsis pennata and found in the mollusk Elysia rufescens (Miguel-Lillo et al., 2015; Sable et al., 2017). It advanced through five clinical trials and completed the safety evaluation in phase I in patients with distinct advanced solid tumors. Nevertheless, kahalalide F dropped in phase II due to lack of efficacy, short half-life, limited spectrum of activity and a poor response in patients. However, due the high potential of this compound as cytotoxic, it inspired the development of several synthetic analogs to overcome its limitations increasing its potency and half-life time (Wang B. et al., 2015).
In the last years nanotechnology has emerged as a promising solution to be used in drug delivery systems to suppress some of these limitations, being considered as one of the next-generation platform for cancer therapy (Sun et al., 2014; Xin et al., 2017). Therefore the production of nano-formulations of drugs derived from algae-derived compounds can be an interesting approach to potentiate anticancer properties. In addition the use of nano-formulations can also be useful to overcome some limitations that arise from specific characteristics of each compound, such as nonspecific biodistribution, low water solubility, lack of targeting capability, systemic toxicity, weak therapeutic effect, and limited bioavailability (Sun et al., 2014; Blanco et al., 2015). For instance one of the most promising compounds isolated from algae with interesting antitumor proprieties is fucoxanthin. However, the poor solubility, chemical instability, and low bioavailability of this carotenoid limit its use in cancer therapeutics. To overcome these drawbacks several approaches have been assessed such as the inclusion of fucoxanthin in nano-emulsions (Huang et al., 2017), nano-suspensions (Muthuirulappan and Francis, 2013) and nano-gels (Ravi and Baskaran, 2015). However as described by Bajpai et al. (2018) for the microalgae, the published data on nano-formulations using compounds derived from macroalgae is also scarce suggesting a new area to be explored that can potentiate the capacity of these compounds in cancer therapeutics.
According to the interesting activities mediated by algae-derived compounds, it is expected that in the next few years some of them may reach the clinical trials stages or inspire the development of new compounds allowing their translation into clinically useful drugs in the future. Moreover, the use of some those compounds as co-adjuvant in pre-existent therapeutics regimes appears to be a valid approach to improve the therapeutic effects of the antitumor drugs and decrease their side-effects.
The Therapeutic Potential of Sphaerococcus coronopifolius Compounds—Case Study
Sphaerococcus coronopifolius is a red alga belonging to the Rhodophyta phylum, which is narrow, compressed, two-edged, cartilaginous, scarlet fronds and main axes that are dark brownish-red. The habitat of this species is rarely on rocks in the lower littoral, but it is common in the shallow sublittoral to a 15 m depth. They are distributed in the East Atlantic (Ireland and Britain to Canary Islands) and Mediterranean and Black Seas (Guiry and Guiry, 2015). Since its first chemical analysis in 1976, S. coronopifolius has demonstrated to be an interesting source of brominated cyclic diterpenes, most of them containing one or two bromine atoms (Rodrigues et al., 2015). Although more than 40 compounds have been isolated and described from S. coronopifolius, in the last four decades, few studies characterized their biological activities. According to previous studies, some of the compounds isolated evidenced great biological activities, including antifouling (Piazza et al., 2011), antimalarial (Etahiri et al., 2001) and antimicrobial (Etahiri et al., 2001; Smyrniotopoulos et al., 2010b,c; Rodrigues et al., 2015). Another interesting bioactivity exhibited by these compounds is their antitumor potential. Several compounds revealed interesting cytotoxic activities in different in vitro models (Table 2). For example, 14R-Hydroxy-13,14-dihydro-sphaerococcenol-A decreased significantly the viability of NSCLC-N6-L16 and A549 human lung cancer cell lines with an IC50 of 5 and 4 μg/mL, respectively. Moreover, spirosphaerol and corfusphaeroxide showed moderate cytotoxic activity against the malignant cell lines A549, Hs683 and MCF-7. Smyrniotopoulos et al. (2010a) isolated several metabolites and evaluated their cytotoxic activity toward four human apoptosis-resistant (U373, A549, SK-MEL-28, OE21) and two human apoptosis-sensitive (PC-3, LoVo) cancer cell lines with IC50 values ranging from 3 to 100 μM. In a study performed by Rodrigues et al. (2015), the compound Sphaerococcenol A exhibited the highest anti-proliferative activity on HepG2 cells with an IC50 of 42.87 μM, being more potent than cisplatin (75.41 μM). Although some compounds isolated from Sphaerococcus coronopifolius have exhibited interesting biological activities, its potential remains understudied since the intracellular mechanisms associated with the observed effects are yet uncovered. S. coronopifolius is therefore a clearly example of the potential of algae as source of cytotoxic compounds, still under explored, suggesting that the potential of algae as source of compounds for the development of new antitumor drugs is probably much higher than previously thought.
Conclusions and Final Remarks
Over the last several decades, marine organisms revealed to be an interesting source of both pre-existing and unrecognized compounds with the potential for providing sustainable economic and human benefits. Many of these compounds demonstrated great potential for therapeutic applications, exhibiting specific and potent activities against different diseases, including cancer. Their potential as a source of antitumor drugs has been proven by the current pipeline. Six of nine drugs from marine origin currently in the market are used in cancer treatment, and several compounds originated or derived from marine organisms are undergoing clinical trials with indications for oncologic therapeutics. However, the number of compounds in the market or in clinical trials is very low compared with the total number of isolated compounds with antitumor potential. This is mainly due to the different constraints between discovery and commercialization such as the discontinuation of “candidates” in clinical trials or preclinical tests due to difficulties on harvesting the organism and on the isolation and purification procedures, low yields, insufficient investment by pharmaceutical companies, environmental policies, high toxicity and low efficiency of the active compounds. Nevertheless, considering the approved marine drugs, it is also possible to observe that six of them were approved in the last 10 years. This fact is probably directly associated with the development of high-throughput screening technologies that allowed faster and more accurate results. Thus, it is expected that the number of antitumor drugs of marine origin will increase in the next few years.
Among the marine organisms, many crude extracts, enriched fractions and compounds obtained from algae displayed interesting antitumor potential along the years. These effects are mediated by compounds from different chemical classes including polysaccharides, terpenoids, phenolic compounds, glicoproteins, sterols, carotenoids, alkaloids, sulfolipids, chlorophylls, and fatty acids. Despite the high number of reports evidencing the cytotoxic and cytostatic properties of algae-derived compounds, few studies have characterized the intracellular signaling pathways underlying their effects. This view is clearly evident with Sphaerococcus coronopifolius, since some of its bioactive compounds exhibited interesting cytotoxic activities on different cell lines, but the intracellular signaling pathways involved in its activities have still not been deeply characterized. Thus, there is a need to design and perform studies to evaluate these type of molecules in more complex biological systems, including in vivo models. Another important tool that still remain unexplored is the use of 3D chemical structural modeling techniques to find new biochemical targets for the algae-derived compounds. Moreover, the idea of using algae-derived compounds as co-adjuvants in therapeutics should also be evaluated.
One of the major challenges in this area is the sustainable production of these compounds to “supply” sufficient quantity for preclinical, clinical and future commercialization, since the algae's slow growth and seasonality together with low extraction yields are significant limitations. In line with this requirement, total chemical synthesis can not only guarantee the sustainable and continuous production of the bioactive molecules but also improve or enhance their functional zones. Aquaculture can also be an interesting approach for the continuous supply of algae. However, more studies are needed to understand if algae continue to produce the desired compounds under artificial conditions.
The applications of new techniques such as nanotechnology to develop new nano-formulations can potentiate the development of anticancer drugs from algae origin leading to the suppression of limitations and improve the capabilities of some algae-derived compounds. However further technologies are needed to validate the improvement of their antitumor activities when applied in nanoformulations. According with this search this area was not yet explored. By other side, the creation/ establishment of interdisciplinary teams (including for example chemists, pharmaceuticals, biotechnologists and biologists) can contribute a faster bioscreening process, from the isolation and identification of algae-derived compounds to full validation of their antitumor capabilities. The studies gathered in the present review clearly demonstrate that algae have great potential as a source of antitumor compounds with the capacity to hamper different key pathway targets involved in cancer development including cell cycle regulation, apoptosis, angiogenesis, migration and invasion processes. Even though, to date algae have not been fully exploited, it is expected that in a few years, their translation to clinical use can be a reality.
Author Contributions
CA and RP conceived the research topic and the design the review. CA, JS, and SP did the bibliographic research. HG and MA critically contributed to discuss the cancer threat and current marine clinical pipeline. LB critically contributed to discuss the antitumor potential of marine algae-derived compounds. All authors performed the literature review and participated in the drafting and revision of the manuscript, thus making a direct and intellectual contribution to the work.
Funding
Authors would like to acknowledge the financial support of the Portuguese Foundation for Science and Technology (FCT) through Strategic Projects UID/MAR/04292/2013 and UID/Multi/04046/2013 granted to MARE – Marine and Environmental Sciences Centre and BioISI - BioSystems and Integrative Sciences Institute, respectively, through Red2Discovery Project (PTDC/MAR-BIO/6149/2014), co-financed by COMPETE (POCI-01-0145-FEDER-016791), and through Oncologia de Precisão: Terapias e Tecnologias Inovadoras project (POINT4PAC) (SAICTPAC/0019/2015 - LISBOA-01-0145-FEDER-016405). The authors also wish to acknowledge the Integrated Programme of SR&TD Smart Valorization of Endogenous Marine Biological Resources Under a Changing Climate (reference Centro-01-0145-FEDER-000018), co-funded by Centro 2020 program, Portugal 2020, European Union, through the European Regional Development Fund. CA, SP, and JS are financial supported by a grant from FCT (SFRH/BD/97764/2013, SFRH/BD/96203/2013, and SFRH/BD/103255/2014, respectively).
Conflict of Interest Statement
The authors declare that the research was conducted in the absence of any commercial or financial relationships that could be construed as a potential conflict of interest.
References
Abu, R., Jiang, Z., Ueno, M., Isaka, S., Nakazono, S., Okimura, T., et al. (2015). Anti-metastatic effects of the sulfated polysaccharide ascophyllan isolated from Ascophyllum nodosum on B16 melanoma. Biochem. Biophys. Res. Commun. 458, 727–732. doi: 10.1016/j.bbrc.2015.01.061
Agrawal, S., Adholeya, A., Barrow, C. J., and Deshmukh, S. K. (2018). Marine fungi: an untapped bioresource for future cosmeceuticals. Phytochem. Lett. 23, 15–20. doi: 10.1016/j.phytol.2017.11.003
Aguilar-Briseño, J., Cruz-Suarez, L., Sassi, J.-F., Ricque-Marie, D., Zapata-Benavides, P., Mendoza-Gamboa, E., et al. (2015). Sulphated polysaccharides from Ulva clathrata and Cladosiphon okamuranus seaweeds both inhibit viral attachment/entry and cell-cell fusion, in NDV infection. Mar. Drugs 13:697. doi: 10.3390/md13020697
Alves, C., Pinteus, S., Horta, A., and Pedrosa, R. (2016a). High cytotoxicity and anti-proliferative activity of algae extracts on an in vitro model of human hepatocellular carcinoma. SpringerPlus 5:1339. doi: 10.1186/s40064-016-2938-2
Alves, C., Pinteus, S., Simões, T., Horta, A., Silva, J., Tecelão, C., et al. (2016b). Bifurcaria bifurcata: a key macro-alga as a source of bioactive compounds and functional ingredients. Int. J. Food Sci. Technol. 51, 1638–1646. doi: 10.1111/ijfs.13135
American Cancer Society (2015). Global Cancer Facts & Figures. Atlanta, GA: American Cancer Society.
AndisInsight (2018). Database for Drug Research and Development, Disease Treatment and Decision Making [Online]. Springer International Publishing AG. Available online at: http://adisinsight.springer.com/ [Accessed 5th January 2018].
Arizza, V. (2013). Marine biodiversity as source of new drugs. Ital. J. Zool. 80, 317–318. doi: 10.1080/11250003.2013.830370
Atkins, J. P., Burdon, D., Elliott, M., and Gregory, A. J. (2011). Management of the marine environment: integrating ecosystem services and societal benefits with the DPSIR framework in a systems approach. Mar. Pollut. Bull. 62, 215–226. doi: 10.1016/j.marpolbul.2010.12.012
Avila, C., Taboada, S., and Núñez-Pons, L. (2008). Antarctic marine chemical ecology: what is next? Mar. Ecol. 29, 1–71. doi: 10.1111/j.1439-0485.2007.00215.x
Bajpai, V. K., Shukla, S., Kang, S. M., Hwang, S. K., Song, X., Huh, Y. S., et al. (2018). Developments of cyanobacteria for nano-marine drugs: relevance of nanoformulations in cancer therapies. Mar. Drugs 16, 179. doi: 10.3390/md16060179
Berkov, S., Mutafova, B., and Christen, P. (2014). Molecular biodiversity and recent analytical developments: a marriage of convenience. Biotechnol. Adv. 32, 1102–1110. doi: 10.1016/j.biotechadv.2014.04.005
Blanco, E., Shen, H., and Ferrari, M. (2015). Principles of nanoparticle design for overcoming biological barriers to drug delivery. Nat. Biotechnol. 33, 941–951. doi: 10.1038/nbt.3330
Blunt, J. W., Carroll, A. R., Copp, B. R., Davis, R. A., Keyzers, R. A., and Prinsep, M. R. (2018). Marine natural products. Nat. Prod. Rep. 35, 8–53. doi: 10.1039/C7NP00052A
Blunt, J. W., Copp, B. R., Hu, W.-P., Munro, M. H., Northcote, P. T., and Prinsep, M. R. (2009). Marine natural products. Nat. Prod. Rep. 26, 170–244. doi: 10.1039/b805113p
Blunt, J. W., Copp, B. R., Hu, W.-P., Munro, M., Northcote, P. T., and Prinsep, M. R. (2007). Marine natural products. Nat. Prod. Rep. 24, 31–86. doi: 10.1039/b603047p
Blunt, J. W., Copp, B. R., Hu, W.-P., Munro, M., Northcote, P. T., and Prinsep, M. R. (2008). Marine natural products. Nat. Prod. Rep. 25, 35–94. doi: 10.1039/b701534h
Blunt, J. W., Copp, B. R., Keyzers, R. A., Munro, M. H. G., and Prinsep, M. R. (2013). Marine natural products. Nat. Prod. Rep. 30, 237–323. doi: 10.1039/C2NP20112G
Blunt, J. W., Copp, B. R., Keyzers, R. A., Munro, M. H. G., and Prinsep, M. R. (2014). Marine natural products. Nat. Prod. Rep. 31, 160–258. doi: 10.1039/c3np70117d
Blunt, J. W., Copp, B. R., Keyzers, R. A., Munro, M. H. G., and Prinsep, M. R. (2015). Marine natural products. Nat. Prod. Rep. 32, 116–211. doi: 10.1039/C4NP00144C
Blunt, J. W., Copp, B. R., Keyzers, R. A., Munro, M. H. G., and Prinsep, M. R. (2016). Marine natural products. Nat. Prod. Rep. 33, 382–431. doi: 10.1039/C5NP00156K
Blunt, J. W., Copp, B. R., Keyzers, R. A., Munro, M. H. G., and Prinsep, M. R. (2017). Marine natural products. Nat. Prod. Rep. 34, 235–294. doi: 10.1039/C6NP00124F
Blunt, J. W., Copp, B. R., Keyzers, R. A., Munro, M. H., and Prinsep, M. R. (2012). Marine natural products. Nat. Prod. Rep. 29, 144–222. doi: 10.1039/C2NP00090C
Blunt, J. W., Copp, B. R., Munro, M. H. G., Northcote, P. T., and Prinsep, M. R. (2003). Marine natural products. Nat. Prod. Rep. 20, 1–48. doi: 10.1039/b207130b
Blunt, J. W., Copp, B. R., Munro, M. H., Northcote, P. T., and Prinsep, M. R. (2004). Marine natural products. Nat. Prod. Rep. 21, 1–49. doi: 10.1039/b305250h
Blunt, J. W., Copp, B. R., Munro, M. H., Northcote, P. T., and Prinsep, M. R. (2005). Review: marine natural products. Nat. Prod. Rep. 22, 15–61. doi: 10.1039/b415080p
Blunt, J. W., Copp, B. R., Munro, M. H., Northcote, P. T., and Prinsep, M. R. (2006). Marine natural products. Nat. Prod. Rep. 23, 26–78. doi: 10.1039/b502792f
Blunt, J. W., Copp, B. R., Munro, M. H., Northcote, P. T., and Prinsep, M. R. (2011). Marine natural products. Nat. Prod. Rep. 28, 196–268. doi: 10.1039/C005001F
Blunt, J. W., Copp, B. R., Munro, M., Northcote, P. T., and Prinsep, M. R. (2010). Marine natural products. Nat. Prod. Rep. 27, 165–237. doi: 10.1039/b906091j
Boo, H.-J., Hong, J.-Y., Kim, S.-C., Kang, J.-I., Kim, M.-K., Kim, E.-J., et al. (2013). The anticancer effect of fucoidan in PC-3 prostate cancer cells. Mar. Drugs 11, 2982–2999. doi: 10.3390/md11082982
Botana, L. M., and Alfonso, A. (2015). Phycotoxins: Chemistry and Biochemistry. Chichester: John Wiley & Sons.
Bouga, M., and Combet, E. (2015). Emergence of seaweed and seaweed-containing foods in the UK: focus on labeling, iodine content, toxicity and nutrition. Foods 4:240. doi: 10.3390/foods4020240
Brahmachari, G. (2015). Bioactive Natural Products: Chemistry and Biology. Wheinheim: John Wiley & Sons.
Brown, E. M., Allsopp, P. J., Magee, P. J., Gill, C. I. R., Nitecki, S., Strain, C. R., et al. (2014). Seaweed and human health. Nutr. Rev. 72, 205–216. doi: 10.1111/nure.12091
Bucar, F., Wube, A., and Schmid, M. (2013). Natural product isolation - how to get from biological material to pure compounds. Nat. Prod. Rep. 30, 525–545. doi: 10.1039/c3np20106f
Cafieri, F., Ciminiello, P., Santacroce, C., and Fattorusso, E. (1982). (1S)- 1, 2-Dihydro-1-hydroxybromosphaerol, a minor bromoditerpene from the red alga Sphaerococcus coronopifolius. Phytochemistry 21, 2412–2413. doi: 10.1016/0031-9422(82)85221-7
Cafieri, F., De Napoli, L., Fattorusso, E., and Santacroce, C. (1987). Diterpenes from the red alga Sphaerococcus coronopifolius. Phytochemistry 26, 471–473. doi: 10.1016/S0031-9422(00)81435-1
Cafieri, F., Fattorusso, E., Mayol, L., and Santacroce, C. (1986). Structure of bromotetrasphaerol, a further irregular diterpene from the red alga Sphaerococcus coronopifolius. Tetrahedron 42, 4273–4276. doi: 10.1016/S0040-4020(01)87653-5
Calado, R., Costa Leal, M., Gaspar, H., Santos, S., Marques, A., Nunes, M. L., et al. (2018). “How to succeed in marketing marine natural products for pharmaceutical, cosmetics & nutraceutical markets,” in Grand Challenges in Marine Biotechnology, Springer Series Grand Challenges in Biology and Biotechnology, eds P. H. Rampelotto and A. Trincone (Basel: Springer), 317–403.
Campos, A., Souza, C. B., Lhullier, C., Falkenberg, M., Schenkel, E. P., Ribeiro-do-Valle, R. M., et al. (2012). Anti-tumour effects of elatol, a marine derivative compound obtained from red algae Laurencia microcladia. J. Pharm. Pharmacol. 64, 1146–1154. doi: 10.1111/j.2042-7158.2012.01493.x
Chandini, S. K., Ganesan, P., Suresh, P., and Bhaskar, N. (2008). Seaweeds as a source of nutritionally beneficial compounds-a review. J. Food Sci. Technol. 45, 1–13.
Chen, J., Alberts, I., and Li, X. (2014). Dysregulation of the IGF-I/PI3K/AKT/mTOR signaling pathway in autism spectrum disorders. Int. J. Dev. Neurosci. 35, 35–41. doi: 10.1016/j.ijdevneu.2014.03.006
Choi, Y., Kim, J., Lee, K., Choi, Y.-J., Ye, B.-R., Kim, M.-S., et al. (2017). Tuberatolide B suppresses cancer progression by promoting ROS-mediated inhibition of STAT3 signaling. Mar. Drugs 15:55. doi: 10.3390/md15030055
Cooper, G. M., and Hausman, R. E. (2013). The Cell: A Molecular Approach. Sunderland: Sinauer Associates.
Cragg, G. M., and Newman, D. J. (2009). Nature: a vital source of leads for anticancer drug development. Phytochem. Rev. 8, 313–331. doi: 10.1007/s11101-009-9123-y
Cragg, G. M., and Newman, D. J. (2013). Natural products: a continuing source of novel drug leads. Biochim. Biophys. Acta 1830, 3670–3695. doi: 10.1016/j.zbbagen.2013.02.008
Da Cruz, J. F., Gaspar, H., and Calado, G. (2012). Turning the game around: toxicity in a nudibranch-sponge predator–prey association. Chemoecology 22, 47–53. doi: 10.1007/s00049-011-0097-z
de Rosa, S., de Stefano, S., Scarpelli, P., and Zavodnik, N. (1988). Terpenes from the red alga Sphaerococcus coronopifolius of the north Adriatic Sea. Phytochemistry 27, 1875–1878. doi: 10.1016/0031-9422(88)80468-0
De Souza, É. T., Pereira de Lira, D., Cavalcanti de Queiroz, A., Costa da Silva, D. J., Bezerra de Aquino, A., Campessato Mella, E. A., et al. (2009). The antinociceptive and anti-inflammatory activities of caulerpin, a bisindole alkaloid isolated from seaweeds of the genus Caulerpa. Mar. Drugs 7, 689–704. doi: 10.3390/md7040689
Deng, R., Zhou, B., Guan, B., Chan, G. C.-F., and Shen, J. (2018). Marine algae extract attenuated osteoporosis in OVX mice, enhanced osteogenesis on human mesenchymal stem cells and promoted OPG expression. J. Funct. Foods 40, 229–237. doi: 10.1016/j.jff.2017.10.044
Dias, D. A., Urban, S., and Roessner, U. (2012). A historical overview of natural products in drug discovery. Metabolites 2:303. doi: 10.3390/metabo2020303
Dias, P., Siqueira, J. Jr., Vendruscolo, L., Neiva, T. D. J., Gagliardi, A., Maraschin, M., et al. (2005). Antiangiogenic and antitumoral properties of a polysaccharide isolated from the seaweed Sargassum stenophyllum. Cancer Chemother. Pharmacol. 56, 436–446. doi: 10.1007/s00280-004-0995-7
Dixson, D. L., Abrego, D., and Hay, M. E. (2014). Chemically mediated behavior of recruiting corals and fishes: a tipping point that may limit reef recovery. Science 345, 892–897. doi: 10.1126/science.1255057
Du, B., Zhong, X., Liao, X., Xu, W., Zhou, X., and Xu, S. (2010). A new antitumor arabinopyranoside from Laurencia majuscula induces G2/M cell cycle arrest. Phytother. Res. 24, 1447–1450. doi: 10.1002/ptr.3153
Egan, S., Harder, T., Burke, C., Steinberg, P., Kjelleberg, S., and Thomas, T. (2013). The seaweed holobiont: understanding seaweed–bacteria interactions. FEMS Microbiol. Rev. 37, 462–476. doi: 10.1111/1574-6976.12011
Eitsuka, T., Nakagawa, K., Igarashi, M., and Miyazawa, T. (2004). Telomerase inhibition by sulfoquinovosyldiacylglycerol from edible purple laver (Porphyra yezoensis). Cancer Lett. 212, 15–20. doi: 10.1016/j.canlet.2004.03.019
EMA (2018a). EU Clinical Trials Register [Online]. Available online at: https://www.clinicaltrialsregister.eu/ctr-search/search [Accessed 5th January of 2018].
EMA (2018b). Medicines [Online]. Available online at: http://www.ema.europa.eu/ema/index.jsp?curl=pages/includes/medicines/medicines_landing_page.jsp&mid= [Accessed 5th January of 2018].
Etahiri, S., Bultel-Poncé, V., Caux, C., and Guyot, M. (2001). New bromoditerpenes from the red Alga Sphaerococcus coronopifolius. J. Nat. Prod. 64, 1024–1027. doi: 10.1021/np0002684
Fattorusso, E., Magno, S., Santacroce, C., Sica, D., Di Blasio, B., Pedone, C., et al. (1976). Bromosphaerol, a new bromine containing diterpenoid from the red alga Sphaerococcus coronopifolius. Gazz. Chim. Ital. 106, 779–783.
Faulkner, D. (1984). Marine natural products: metabolites of marine invertebrates. Nat. Prod. Rep. 1, 551–598. doi: 10.1039/np9840100551
Faulkner, D. (1993). Marine natural products. Nat. Prod. Rep. 10, 497–539. doi: 10.1039/np9931000497
Faulkner, D. (1994). Marine natural products. Nat. Prod. Rep. 11, 355–394. doi: 10.1039/np9941100355
Faulkner, D. (1995). Marine natural products. Nat. Prod. Rep. 12, 223–269. doi: 10.1039/np9951200223
Faulkner, D. J. (1996). Marine natural products. Nat. Prod. Rep. 13, 75–125. doi: 10.1039/np9961300075
Faulkner, D. J. (1997). Marine natural products. Nat. Prod. Rep. 14, 259–302. doi: 10.1039/np9971400259
FDA (2018a). ClinicalTrials.gov [Online]. Available online at: https://clinicaltrials.gov/ [Accessed 5th January 2018].
FDA (2018b). Drugs@FDA: FDA Approved Drug Products [Online]. Available online at: http://www.accessdata.fda.gov/scripts/cder/daf/ [Accessed 5th January of 2018].
Fenical, W., Finer, J., and Clardy, J. (1976). Sphaerococcenol A; a new rearranged bromo-diterpene from the red alga Sphaerococcus coronopifolius. Tetrahedron. Lett. 17, 731–734. doi: 10.1016/S0040-4039(00)77936-6
Fischel, J., Lemee, R., Formento, P., Caldani, C., Moll, J., Pesando, D., et al. (1994). Cell growth inhibitory effects of caulerpenyne, a sesquiterpenoid from the marine algae Caulerpa taxifolia. Anticancer Res. 15, 2155–2160.
Fukuda, Y., Sugahara, T., Ueno, M., Fukuta, Y., Ochi, Y., Akiyama, K., et al. (2006). The anti-tumor effect of Euchema serra agglutinin on colon cancer cells in vitro and in vivo. Anti-Cancer Drugs 17, 943–947. doi: 10.1097/01.cad.0000224458.13651.b4
Furuno, A., Watari, K., Nakamura, M., Fukunaga, Y., Jung, J. H., and Ono, M. (2011). Natural anti-inflammatory enone fatty acid inhibits angiogenesis by attenuating nuclear factor-κB signaling in vascular endothelial cells. Int. J. Oncol. 38, 493–501. doi: 10.3892/ijo.2010.856
Ganesan, P., Noda, K., Manabe, Y., Ohkubo, T., Tanaka, Y., Maoka, T., et al. (2011). Siphonaxanthin, a marine carotenoid from green algae, effectively induces apoptosis in human leukemia (HL-60) cells. Biochim. Biophys. Acta 1810, 497–503. doi: 10.1016/j.bbagen.2011.02.008
Go, H., Hwang, H.-J., and Nam, T.-J. (2010). A glycoprotein from Laminaria japonica induces apoptosis in HT-29 colon cancer cells. Toxicol. In Vitro 24, 1546–1553. doi: 10.1016/j.tiv.2010.06.018
Gotsbacher, M., and Karuso, P. (2015). new antimicrobial bromotyrosine analogues from the sponge Pseudoceratina purpurea and its predator Tylodina corticalis. Mar. Drugs 13:1389. doi: 10.3390/md13031389
Graça, A. P., Bondoso, J., Gaspar, H., Xavier, J. R., Monteiro, M. C., de la Cruz, M., et al. (2013). Antimicrobial activity of heterotrophic bacterial communities from the marine sponge Erylus discophorus (Astrophorida, Geodiidae). PLoS ONE 8:e78992. doi: 10.1371/journal.pone.0078992
Gribble, G. (2015). Biological activity of recently discovered halogenated marine natural products. Mar. Drugs 13:4044. doi: 10.3390/md13074044
Gross, H., Goeger, D. E., Hills, P., Mooberry, S. L., Ballantine, D. L., Murray, T. F., et al. (2006). Lophocladines, bioactive alkaloids from the red alga Lophocladia sp. J. Nat. Prod. 69, 640–644. doi: 10.1021/np050519e
Guerra Dore, C. M. P., Faustino Alves, M. G. C., Santos, N. D., Cruz, A. K. M., Câmara, R. B. G., Castro, A. J. G., et al. (2013). Antiangiogenic activity and direct antitumor effect from a sulfated polysaccharide isolated from seaweed. Microvasc. Res. 88, 12–18. doi: 10.1016/j.mvr.2013.03.001
Guiry, M. D., and Guiry, G. M. (2015). AlgaeBase. World-wide electronic publication, National University of Ireland, Galway [Online]. Galway: National University of Ireland. Available online at: http://www.algaebase.org [Accessed 5th of January of 2018].
Haefner, B. (2003). Drugs from the deep: marine natural products as drug candidates. Drug Discov. Today 8, 536–544. doi: 10.1016/S1359-6446(03)02713-2
Halpern, B. S., Longo, C., Hardy, D., McLeod, K. L., Samhouri, J. F., Katona, S. K., et al. (2012). An index to assess the health and benefits of the global ocean. Nature 488, 615–620. doi: 10.1038/nature11397
Hanahan, D., and Weinberg, R. A. (2000). The hallmarks of cancer. Cell 100, 57–70. doi: 10.1016/S0092-8674(00)81683-9
Hanahan, D., and Weinberg, R. A. (2011). Hallmarks of cancer: the next generation. Cell 144, 646–674. doi: 10.1016/j.cell.2011.02.013
Hay, M. E. (2009). Marine chemical ecology: chemical signals and cues structure marine populations, communities, and ecosystems. Annu. Rev. Mar. Sci. 1, 193–212. doi: 10.1146/annurev.marine.010908.163708
Heo, S.-J., Kim, K.-N., Yoon, W.-J., Oh, C., Choi, Y.-U., Affan, A., et al. (2011). Chromene induces apoptosis via caspase-3 activation in human leukemia HL-60 cells. Food Chem. Toxicol. 49, 1998–2004. doi: 10.1016/j.fct.2011.05.011
Horta, A., Pinteus, S., Alves, C., Fino, N., Silva, J., Fernandez, S., et al. (2014). Antioxidant and antimicrobial potential of the Bifurcaria bifurcata epiphytic bacteria. Mar. Drugs 12, 1676–1689. doi: 10.3390/md12031676
Housman, G., Byler, S., Heerboth, S., Lapinska, K., Longacre, M., Snyder, N., et al. (2014). Drug resistance in cancer: an overview. Cancers 6:1769. doi: 10.3390/cancers6031769
Huang, Z., Xu, L., Zhu, X., Hu, J., Peng, H., Zeng, Z., et al. (2017). Stability and bioaccessibility of fucoxanthin in nanoemulsions prepared from pinolenic acid-contained structured lipid. Int. J. Food Eng. 13, 14. doi: 10.1515/ijfe-2016-0273
Ianora, A., Boersma, M., Casotti, R., Fontana, A., Harder, J., Hoffmann, F., et al. (2006). New trends in marine chemical ecology. Estuar. Coast. 29, 531–551. doi: 10.1007/BF02784281
Jiao, L., Li, X., Li, T., Jiang, P., Zhang, L., Wu, M., et al. (2009). Characterization and anti-tumor activity of alkali-extracted polysaccharide from Enteromorpha intestinalis. Int. Immunopharmacol. 9, 324–329. doi: 10.1016/j.intimp.2008.12.010
Kang, S.-M., Kim, A.-D., Heo, S.-J., Kim, K.-N., Lee, S.-H., Ko, S.-C., et al. (2012). Induction of apoptosis by diphlorethohydroxycarmalol isolated from brown alga, Ishige okamurae. J. Funct. Foods 4, 433–439. doi: 10.1016/j.jff.2012.02.001
Kawaguchi, T., Hayakawa, M., Koga, H., and Torimura, T. (2015). Effects of fucoidan on proliferation, AMP-activated protein kinase, and downstream metabolism- and cell cycle-associated molecules in poorly differentiated human hepatoma HLF cells. Int. J. Oncol. 46, 2216–2222. doi: 10.3892/ijo.2015.2928
Ke, Q., and Costa, M. (2006). Hypoxia-Inducible Factor-1 (HIF-1). Mol. Pharmacol. 70, 1469–1480. doi: 10.1124/mol.106.027029
Kicklighter, C. E., Kamio, M., Nguyen, L., Germann, M. W., and Derby, C. D. (2011). Mycosporine-like amino acids are multifunctional molecules in sea hares and their marine community. Proc. Natl. Acad. Sci. U.S.A. 108, 11494–11499. doi: 10.1073/pnas.1103906108
Kim, A., Lee, Y., Kang, S.-H., Kim, G., Kim, H., and Hyun, J. (2013). cytotoxic effect of clerosterol isolated from Codium fragile on A2058 human melanoma cells. Mar. Drugs 11:418. doi: 10.3390/md11020418
Kim, I., Choi, J., Lee, M., Kwon, C., and Nam, T. (2018). Anti-obesity effects of pectinase and cellulase enzyme-treated Ecklonia cava extract in high-fat diet-fed C57BL/6N mice. Int. J. Mol. Med. 41 924–934. doi: 10.3892/ijmm.2017.3295
Kim, S.-K. (2014). Seafood Processing By-Products -Trends and Applications. New York, NY: Springer-Verlag.
Kong, C.-S., Kim, J.-A., Yoon, N.-Y., and Kim, S.-K. (2009). Induction of apoptosis by phloroglucinol derivative from Ecklonia cava in MCF-7 human breast cancer cells. Food Chem. Toxicol. 47, 1653–1658. doi: 10.1016/j.fct.2009.04.013
Kubanek, J., Prusak, A. C., Snell, T. W., Giese, R. A., Hardcastle, K. I., Fairchild, C. R., et al. (2005). antineoplastic diterpene–benzoate macrolides from the fijian red alga Callophycus serratus. Org. Lett. 7, 5261–5264. doi: 10.1021/ol052121f
Kwak, J.-Y. (2014). Fucoidan as a marine anticancer agent in preclinical development. Mar. Drugs 12, 851–870. doi: 10.3390/md12020851
Kwon, M.-J., and Nam, T.-J. (2007). A polysaccharide of the marine alga Capsosiphon fulvescens induces apoptosis in AGS gastric cancer cells via an IGF-IR-mediated PI3K/Akt pathway. Cell Biol. Int. 31, 768–775. doi: 10.1016/j.cellbi.2007.01.010
Leal, M. C., Munro, M. H. G., Blunt, J. W., Puga, J., Jesus, B., Calado, R., et al. (2013). Biogeography and biodiscovery hotspots of macroalgal marine natural products. Nat. Prod. Rep. 30, 1380–1390. doi: 10.1039/c3np70057g
Lee, J., Kim, S., Jung, W.-S., Song, D.-G., Um, B.-H., Son, J.-K., et al. (2012). Phlorofucofuroeckol-A, a potent inhibitor of aldo-keto reductase family 1 member B10, from the edible brown alga Eisenia bicyclis. J. Korean Soc. Appl. Biol. Chem. 55, 721–727. doi: 10.1007/s13765-012-2169-3
Lee, J.-H., Park, S., Hossain, M., Kim, M., Kim, M.-N., Chung, H., et al. (2007). 2,3,6- Tribromo- 4,5- dihydroxybenzyl methyl ether induces growth inhibition and apoptosis in MCF-7 human breast cancer cells. Arch. Pharm. Res. 30, 1132–1137. doi: 10.1007/BF02980248
Li, Y.-X., Li, Y., Qian, Z.-J., Ryu, B., and Kim, S.-K. (2011). Suppression of vascular endothelial growth factor (VEGF) induced angiogenic responses by fucodiphloroethol G. Process Biochem. 46, 1095–1103. doi: 10.1016/j.procbio.2011.01.035
Lins, K. O. A. L., Bezerra, D. P., Alves, A. P. N. N., Alencar, N. M. N., Lima, M. W., Torres, V. M., et al. (2009). Antitumor properties of a sulfated polysaccharide from the red seaweed Champia feldmannii (Diaz-Pifferer). J. Appl. Toxicol. 29, 20–26. doi: 10.1002/jat.1374
Liu, C.-L., Lim, Y.-P., and Hu, M.-L. (2013). Fucoxanthin enhances cisplatin-induced cytotoxicity via NFκB-mediated pathway and downregulates DNA repair gene expression in human hepatoma HepG2 cells. Mar. Drugs 11:50. doi: 10.3390/md11010050
Liu, M., Zhang, W., Wei, J., Qiu, L., and Lin, X. (2012). Marine bromophenol bis(2,3-dibromo-4,5-dihydroxybenzyl) ether, induces mitochondrial apoptosis in K562 cells and inhibits topoisomerase I in vitro. Toxicol. Lett. 211, 126–134. doi: 10.1016/j.toxlet.2012.03.771
Liu, Y., Morgan, J. B., Coothankandaswamy, V., Liu, R., Jekabsons, M. B., Mahdi, F., et al. (2009). The caulerpa pigment caulerpin inhibits HIF-1 activation and mitochondrial respiration. J. Nat. Prod. 72, 2104–2109. doi: 10.1021/np9005794
Lordan, S., Ross, R. P., and Stanton, C. (2011). Marine bioactives as functional food ingredients: potential to reduce the incidence of chronic diseases. Mar. Drugs 9:1056. doi: 10.3390/md9061056
Ludwig, M., Enzenhofer, E., Schneider, S., Rauch, M., Bodenteich, A., Neumann, K., et al. (2013). Efficacy of a Carrageenan nasal spray in patients with common cold: a randomized controlled trial. Respir. Res. 14:124. doi: 10.1186/1465-9921-14-124
Maeda, H., Hosokawa, M., Sashima, T., Funayama, K., and Miyashita, K. (2005). Fucoxanthin from edible seaweed, Undaria pinnatifida, shows antiobesity effect through UCP1 expression in white adipose tissues. Biochem. Biophys. Res. Commun. 332, 392–397. doi: 10.1016/j.bbrc.2005.05.002
Magalhaes, K. D., Costa, L. S., Fidelis, G. P., Oliveira, R. M., Nobre, L. T. D. B., Dantas-Santos, N., et al. (2011). Anticoagulant, antioxidant and antitumor activities of heterofucans from the seaweed Dictyopteris delicatula. Int. J. Mol. Sci. 12:3352. doi: 10.3390/ijms12053352
Mahdi, F., Falkenberg, M., Ioannou, E., Roussis, V., Zhou, Y.-D., and Nagle, D. G. (2011). Thyrsiferol inhibits mitochondrial respiration and HIF-1 activation. Phytochem. Lett. 4, 75–78. doi: 10.1016/j.phytol.2010.09.003
Margulis, L., and Chapman, M. J. (2009). Kingdoms and Domains: An Illustrated Guide to the Phyla of Life on Earth. London: Academic Press.
Marín-Ramos, N. I., Alonso, D., Ortega-Gutiérrez, S., Ortega-Nogales, F. J., Balabasquer, M., Vázquez-Villa, H., et al. (2015). New inhibitors of angiogenesis with antitumor activity in vivo. J. Med. Chem. 58, 3757–3766. doi: 10.1021/jm5019252
Martins, A., Vieira, H., Gaspar, H., and Santos, S. (2014). Marketed marine natural products in the pharmaceutical and cosmeceutical industries: tips for success. Mar. Drugs 12:1066. doi: 10.3390/md12021066
Mayer, A. M. S. (2018). Marine Pharmaceuticals: The Clinical Pipeline. [Online]. Available online at: http://marinepharmacology.midwestern.edu/clinPipeline.htm [Accessed 5th January of 2018].
Mayer, A., Rodríguez, A., Taglialatela-Scafati, O., and Fusetani, N. (2013). Marine pharmacology in 2009–2011: marine compounds with antibacterial, antidiabetic, antifungal, anti-inflammatory, antiprotozoal, antituberculosis, and antiviral activities; affecting the immune and nervous systems, and other miscellaneous mechanisms of action. Mar. Drugs 11:2510. doi: 10.3390/md11072510
Mayer, A., Rodríguez, A., Taglialatela-Scafati, O., and Fusetani, N. (2017). Marine pharmacology in 2012–2013: marine compounds with antibacterial, antidiabetic, antifungal, anti-inflammatory, antiprotozoal, antituberculosis, and antiviral activities; affecting the immune and nervous systems, and other miscellaneous mechanisms of action. Mar. Drugs 15:273. doi: 10.3390/md15090273
Miguel-Lillo, B., Valenzuela, B., Peris-Ribera, J. E., Soto-Matos, A., and Pérez-Ruixo, J. J. (2015). Population pharmacokinetics of kahalalide F in advanced cancer patients. Cancer chemother. Pharmacol. 76, 365–374. doi: 10.1007/s00280-015-2800-1
Mishra, B. B., and Tiwari, V. K. (2011). Natural products: an evolving role in future drug discovery. Eur. J. Med. Chem. 46, 4769–4807. doi: 10.1016/j.ejmech.2011.07.057
Moghadamtousi, S. Z., Karimian, H., Khanabdali, R., Razavi, M., Firoozinia, M., Zandi, K., et al. (2014). Anticancer and antitumor potential of fucoidan and fucoxanthin, two main metabolites isolated from brown algae. Sci. World J. 2014:768323. doi: 10.1155/2014/768323
Mohamed, S., Hashim, S. N., and Rahman, H. A. (2012). Seaweeds: a sustainable functional food for complementary and alternative therapy. Trends Food Sci. Technol. 23, 83–96. doi: 10.1016/j.tifs.2011.09.001
Mohammed, K. A., Hossain, C. F., Zhang, L., Bruick, R. K., Zhou, Y.-D., and Nagle, D. G. (2004). Laurenditerpenol, a new diterpene from the tropical marine alga Laurencia intricata that potently inhibits HIF-1 Mediated hypoxic signaling in breast tumor cells. J. Nat. Prod. 67, 2002–2007. doi: 10.1021/np049753f
Molinski, T. F., Dalisay, D. S., Lievens, S. L., and Saludes, J. P. (2009). Drug development from marine natural products. Nat. Rev. Drug. Discov. 8, 69–85. doi: 10.1038/nrd2487
Moon, S.-M., Lee, S. A., Han, S. H., Park, B.-R., Choi, M. S., Kim, J.-S., et al. (2018). Aqueous extract of Codium fragile alleviates osteoarthritis through the MAPK/NF-κB pathways in IL-1β-induced rat primary chondrocytes and a rat osteoarthritis model. Biomed. Pharmacother. 97, 264–270. doi: 10.1016/j.biopha.2017.10.130
Mora, C., Tittensor, D. P., Adl, S., Simpson, A. G. B., and Worm, B. (2011). How many species are there on earth and in the ocean? PLoS Biol. 9:e1001127. doi: 10.1371/journal.pbio.1001127
Moussavou, G., Kwak, D., Obiang-Obonou, B., Maranguy, C., Dinzouna-Boutamba, S.-D., Lee, D., et al. (2014). Anticancer effects of different seaweeds on human colon and breast cancers. Mar. Drugs 12:4898. doi: 10.3390/md12094898
Murad, H., Ghannam, A., Al-Ktaifani, M., Abbas, A., and Hawat, M. (2015). Algal sulfated carrageenan inhibits proliferation of MDA-MB-231 cells via apoptosis regulatory genes. Mol. Med. Rep. 11, 2153–2158. doi: 10.3892/mmr.2014.2915
Murphy, C., Hotchkiss, S., Worthington, J., and McKeown, S. R. (2014). The potential of seaweed as a source of drugs for use in cancer chemotherapy. J. Appl. Phycol. 26, 2211–2264. doi: 10.1007/s10811-014-0245-2
Murray, P. M., Moane, S., Collins, C., Beletskaya, T., Thomas, O. P., Duarte, A. W. F., et al. (2013). Sustainable production of biologically active molecules of marine based origin. New Biotechnol. 30, 839–850. doi: 10.1016/j.nbt.2013.03.006
Muthuirulappan, S., and Francis, S. P. (2013). Anti-cancer mechanism and possibility of nano-suspension formulation for a marine algae product fucoxanthin. Asian Pac. J. Cancer Prev. 14, 2213–2216. doi: 10.7314/APJCP.2013.14.4.2213
Newman, D. J., and Cragg, G. M. (2012). Natural products as sources of new drugs over the 30 years from 1981 to 2010. J. Nat. Prod. 75, 311–335. doi: 10.1021/np200906s
Newman, D. J., Cragg, G. M., and Battershill, C. N. (2009). Therapeutic agents from the sea: biodiversity, chemo-evolutionary insight and advances to the end of Darwin's 200th year. Diving Hyperb. Med. 39, 216–225.
Nguyen, V., Qian, Z.-J., Lee, B., Heo, S.-J., Kim, K.-N., Jeon, Y.-J., et al. (2014). Fucoxanthin derivatives from Sargassum siliquastrum inhibit matrix metalloproteinases by suppressing NF-kB and MAPKs in human fibrosarcoma cells. Algae 29, 355–366. doi: 10.4490/algae.2014.29.4.355
Nobili, S., Lippi, D., Witort, E., Donnini, M., Bausi, L., Mini, E., et al. (2009). Natural compounds for cancer treatment and prevention. Pharmacol. Res. 59, 365–378. doi: 10.1016/j.phrs.2009.01.017
Noyer, C., Thomas, O. P., and Becerro, M. A. (2011). Patterns of chemical diversity in the mediterranean sponge Spongia lamella. PLoS ONE 6:e20844. doi: 10.1371/journal.pone.0020844
Oh, S.-M., Park, C., Kang, J., Kim, E.-J., Chee, H., Lee, B., et al. (2011). Dieckol inhibits 12-O-tetradecanoylphorbol-13-acetate-induced SK-Hep1 human hepatoma cell motility through suppression of matrix metalloproteinase-9 activity. J. Korean Soc. Appl. Biol. Chem. 54, 376–381. doi: 10.3839/jksabc.2011.059
Pangestuti, R., and Kim, S.-K. (2011). Neuroprotective effects of marine algae. Mar. Drugs. 9, 803–818. doi: 10.3390/md9050803
Park, H., Kim, I., Kim, J., and Nam, T. (2013). Induction of apoptosis and the regulation of ErbB signaling by laminarin in HT-29 human colon cancer cells. Int. J. Mol. Med. 32, 291–295. doi: 10.3892/ijmm.2013.1409
Park, S., and Jeon, Y. (2012). Dieckol from Ecklonia cava suppresses the migration and invasion of HT1080 cells by inhibiting the focal adhesion kinase pathway downstream of Rac1-ROS signaling. Mol. Cell. 33, 141–149. doi: 10.1007/s10059-012-2192-6
Pec, M. K., Aguirre, A., Moser-Thier, K., Fernández, J. J., Souto, M. L., Dorta, J., et al. (2003). Induction of apoptosis in estrogen dependent and independent breast cancer cells by the marine terpenoid dehydrothyrsiferol. Biochem. Pharmacol. 65, 1451–1461. doi: 10.1016/S0006-2952(03)00123-0
Pérez-Recalde, M., Matulewicz, M. C., Pujol, C. A., and Carlucci, M. J. (2014). In vitro and in vivo immunomodulatory activity of sulfated polysaccharides from red seaweed Nemalion helminthoides. Int. J. Biol. Macromol. 63, 38–42. doi: 10.1016/j.ijbiomac.2013.10.024
Piazza, V., Roussis, V., Garaventa, F., Greco, G., Smyrniotopoulos, V., Vagias, C., et al. (2011). Terpenes from the red alga Sphaerococcus coronopifolius inhibit the settlement of barnacles. Mar. Biotechnol. 13, 764–772. doi: 10.1007/s10126-010-9337-4
Pinteus, S., Alves, C., Monteiro, H., Araújo, E., Horta, A., and Pedrosa, R. (2015). Asparagopsis armata and Sphaerococcus coronopifolius as a natural source of antimicrobial compounds. World J. Microbiol. Biotechnol. 31, 445–451. doi: 10.1007/s11274-015-1797-2
Pinteus, S., Silva, J., Alves, C., Horta, A., Fino, N., Rodrigues, A. I., et al. (2017). Cytoprotective effect of seaweeds with high antioxidant activity from the Peniche coast (Portugal). Food Chem. 218, 591–599. doi: 10.1016/j.foodchem.2016.09.067
Plouguerné, E., da Gama, B. A. P., Pereira, R. C., and Barreto-Bergter, E. (2014). Glycolipids from seaweeds and their potential biotechnological applications. Front. Cell. Infect. Microbiol. 4:174. doi: 10.3389/fcimb.2014.00174
Qi, X., Liu, G., Qiu, L., Lin, X., and Liu, M. (2015). Marine bromophenol bis(2,3-dibromo-4,5-dihydroxybenzyl) ether, represses angiogenesis in HUVEC cells and in zebrafish embryos via inhibiting the VEGF signal systems. Biomed. Pharmacother. 75, 58–66. doi: 10.1016/j.biopha.2015.08.033
Rajagopala, S. V., Vashee, S., Oldfield, L. M., Suzuki, Y., Venter, J. C., Telenti, A., et al. (2017). The human microbiome and cancer. Cancer Prev. Res. 10, 226–234. doi: 10.1158/1940-6207.CAPR-16-0249
Rasher, D. B., and Hay, M. E. (2010). Chemically rich seaweeds poison corals when not controlled by herbivores. Proc. Natl. Acad. Sci. U.S.A. 107, 9683–9688. doi: 10.1073/pnas.0912095107
Rasher, D. B., and Hay, M. E. (2014). Competition induces allelopathy but suppresses growth and anti-herbivore defence in a chemically rich seaweed. Proc. R. Soc. Lond. B Biol. Sci. 281:20132615. doi: 10.1098/rspb.2013.2615
Rasher, D. B., Stout, E. P., Engel, S., Kubanek, J., and Hay, M. E. (2011). Macroalgal terpenes function as allelopathic agents against reef corals. Proc. Natl. Acad. Sci. U.S.A. 108, 17726–17731. doi: 10.1073/pnas.1108628108
Ravi, H., and Baskaran, V. (2015). Biodegradable chitosan-glycolipid hybrid nanogels: a novel approach to encapsulate fucoxanthin for improved stability and bioavailability. Food Hydrocoll. 43, 717–725. doi: 10.1016/j.foodhyd.2014.08.004
Rodrigues, D., Alves, C., Horta, A., Pinteus, S., Silva, J., Culioli, G., et al. (2015). Antitumor and antimicrobial potential of bromoditerpenes isolated from the red alga, Sphaerococcus coronopifolius. Mar. Drugs 13, 713–726. doi: 10.3390/md13020713
Rokkaku, T., Kimura, R., Ishikawa, C., Yasumoto, T., Senba, M., Kanaya, F., et al. (2013). Anticancer effects of marine carotenoids, fucoxanthin and its deacetylated product, fucoxanthinol, on osteosarcoma. Int. J. Oncol. 43, 1176–1186. doi: 10.3892/ijo.2013.2019
Ryu, J., Hwang, H.-J., Kim, I.-H., and Nam, T.-J. (2012). Mechanism of inhibition of HepG2 cell proliferation by a glycoprotein from Hizikia fusiformis. Korean J. Fish. Aquat. Sci. 45, 553–560. doi: 10.5657/KFAS.2012.0553
Sable, R., Parajuli, P., and Jois, S. (2017). Peptides, peptidomimetics, and polypeptides from marine sources: a wealth of natural sources for pharmaceutical applications. Mar. Drugs. 15:124. doi: 10.3390/md15040124
Saha, M., Rempt, M., Gebser, B., Grueneberg, J., Pohnert, G., and Weinberger, F. (2012). Dimethylsulphopropionate (DMSP) and proline from the surface of the brown alga Fucus vesiculosus inhibit bacterial attachment. Biofouling 28, 593–604. doi: 10.1080/08927014.2012.698615
Saha, M., Rempt, M., Grosser, K., Pohnert, G., and Weinberger, F. (2011). Surface-associated fucoxanthin mediates settlement of bacterial epiphytes on the rockweed Fucus vesiculosus. Biofouling 27, 423–433. doi: 10.1080/08927014.2011.580841
Santos, S. A. O., Vilela, C., Freire, C. S. R., Abreu, M. H., Rocha, S. M., and Silvestre, A. J. D. (2015). Chlorophyta and Rhodophyta macroalgae: a source of health promoting phytochemicals. Food Chem. 183, 122–128. doi: 10.1016/j.foodchem.2015.03.006
Sathivel, A., Raghavendran, H. R. B., Srinivasan, P., and Devaki, T. (2008). Anti-peroxidative and anti-hyperlipidemic nature of Ulva lactuca crude polysaccharide on d-Galactosamine induced hepatitis in rats. Food Chem. Toxicol. 46, 3262–3267. doi: 10.1016/j.fct.2008.07.016
Sawadogo, W., Boly, R., Cerella, C., Teiten, M., Dicato, M., and Diederich, M. (2015). A survey of marine natural compounds and their derivatives with anti-cancer activity reported in 2012. Molecules 20:7097. doi: 10.3390/molecules20047097
Schulz, W. (2007). Molecular Biology of Human Cancers - Molecular Biology of Human Cancers. Dordrecht: Springer.
Simmons, T. L., Andrianasolo, E., McPhail, K., Flatt, P., and Gerwick, W. H. (2005). Marine natural products as anticancer drugs. Mol. Cancer Ther. 4, 333–342.
Singh, R. P., and Reddy, C. R. K. (2014). Seaweed–microbial interactions: key functions of seaweed-associated bacteria. FEMS Microbiol. Ecol. 88, 213–230. doi: 10.1111/1574-6941.12297
Sivagnanam, S., Yin, S., Choi, J., Park, Y., Woo, H., and Chun, B. (2015). Biological Properties of fucoxanthin in oil recovered from two brown seaweeds using supercritical CO2 extraction. Mar. Drugs 13:3422. doi: 10.3390/md13063422
Skibola, C. (2004). The effect of Fucus vesiculosus, an edible brown seaweed, upon menstrual cycle length and hormonal status in three pre-menopausal women: a case report. BMC Complement. Altern. Med. 4:10. doi: 10.1186/1472-6882-4-10
Smith, T. E., Pond, C. D., Pierce, E., Harmer, Z. P., Kwan, J., Zachariah, M. M., et al. (2018). Accessing chemical diversity from the uncultivated symbionts of small marine animals. Nat. Chem. Biol. 14, 179–185. doi: 10.1038/nchembio.2537
Smyrniotopoulos, V., Kiss, R., Mathieu, V., Vagias, C., and Roussis, V. (2015). Diterpenes with unprecedented skeletons from the red alga Sphaerococcus coronopifolius. Eur. J. Org. Chem. 2015, 2848–2853. doi: 10.1002/ejoc.201500133
Smyrniotopoulos, V., Quesada, A., Vagias, C., Moreau, D., Roussakis, C., and Roussis, V. (2008). Cytotoxic bromoditerpenes from the red alga Sphaerococcus coronopifolius. Tetrahedron 64, 5184–5190. doi: 10.1016/j.tet.2008.03.042
Smyrniotopoulos, V., Vagias, C., Bruyère, C., Lamoral-Theys, D., Kiss, R., and Roussis, V. (2010a). Structure and in vitro antitumor activity evaluation of brominated diterpenes from the red alga Sphaerococcus coronopifolius. Bioorganic Med. Chem. 18, 1321–1330. doi: 10.1016/j.bmc.2009.12.025
Smyrniotopoulos, V., Vagias, C., Rahman, M. M., Gibbons, S., and Roussis, V. (2010b). Ioniols I and II, tetracyclic diterpenes with antibacterial activity, from Sphaerococcus coronopifolius. Chem. Biodivers. 7, 666–676. doi: 10.1002/cbdv.200900026
Smyrniotopoulos, V., Vagias, C., Rahman, M. M., Gibbons, S., and Roussis, V. (2010c). Structure and antibacterial activity of brominated diterpenes from the red alga Sphaerococcus coronopifolius. Chem. Biodivers. 7, 186–195. doi: 10.1002/cbdv.200800309
Smyrniotopoulos, V., Vagias, C., and Roussis, V. (2009). Sphaeroane and Neodolabellane diterpenes from the red alga Sphaerococcus coronopifolius. Mar. Drugs 7:184. doi: 10.3390/md7020184
Spoerner, M., Wichard, T., Bachhuber, T., Stratmann, J., and Oertel, W. (2012). Growth and thallus morphogenesis of Ulva mutabilis (Chlorophyta) depends on a combination of two bacterial species excreting regulatory factors. J. Phycol. 48, 1433–1447. doi: 10.1111/j.1529-8817.2012.01231.x
Sun, T., Zhang, Y. S., Pang, B., Hyun, D. C., Yang, M., and Xia, Y. (2014). Engineered nanoparticles for drug delivery in cancer therapy. Angew. Chem. Int. Edit. 53, 12320–12364. doi: 10.1002/anie.201403036
Svensson, J. R., Nylund, G. M., Cervin, G., Toth, G. B., and Pavia, H. (2013). Novel chemical weapon of an exotic macroalga inhibits recruitment of native competitors in the invaded range. J. Ecol. 101, 140–148. doi: 10.1111/1365-2745.12028
Tang, X., Li, J., Xin, X., and Geng, M. (2006). A new marine-derived sulfated polysaccharide from brown alga suppresses tumor metastasis both in vitro and in vivo. Cancer Biol. Ther. 5, 1474–1480. doi: 10.4161/cbt.5.11.3278
Tarhouni-Jabberi, S., Zakraoui, O., Ioannou, E., Riahi-Chebbi, I., Haoues, M., Roussis, V., et al. (2017). Mertensene, a halogenated monoterpene, induces G2/M cell cycle arrest and caspase dependent apoptosis of human colon adenocarcinoma HT29 cell line through the modulation of ERK-1/-2, AKT and NF-κB signaling. Mar. Drugs 15:221. doi: 10.3390/md15070221
Teas, J., Braverman, L. E., Kurzer, M. S., Pino, S., Hurley, T. G., and Hebert, J. R. (2007). Seaweed and soy: companion foods in asian cuisine and their effects on thyroid function in american women. J. Med. Food 10, 90–100. doi: 10.1089/jmf.2005.056
Thangam, R., Senthilkumar, D., Suresh, V., Sathuvan, M., Sivasubramanian, S., Pazhanichamy, K., et al. (2014). Induction of ROS-dependent mitochondria-mediated intrinsic apoptosis in MDA-MB-231 cells by glycoprotein from Codium decorticatum. J. Agric. Food Chem. 62, 3410–3421. doi: 10.1021/jf405329e
Tocaciu, S., Oliver, L. J., Lowenthal, R. M., Peterson, G. M., Patel, R., Shastri, M., et al. (2018). The effect of Undaria pinnatifida fucoidan on the pharmacokinetics of letrozole and tamoxifen in patients with breast cancer. Integr. Cancer Ther. 17, 99–105. doi: 10.1177/1534735416684014
Torre, L. A., Siegel, R. L., Ward, E. M., and Jemal, A. (2015). Global cancer incidence and mortality rates and trends—an update. Cancer Epidemiol. Biomarkers Prev. 25, 16–27. doi: 10.1158/1055-9965.EPI-15-0578
Tsai, H. L., Tai, C. J., Huang, C. W., Chang, F. R., and Wang, J. Y. (2017). Efficacy of low-molecular-weight fucoidan as a supplemental therapy in metastatic colorectal cancer patients: a double-blind randomized controlled trial. Mar. Drugs. 15, 122. doi: 10.3390/md15040122
Urbano, A. M., Rodrigues, C. F., Cerveira, J. F., Ferreira, L. M. R., and Alpoim, M. C. (2011). “DNA damage, repair and misrepair in cancer and in cancer therapy,” in DNA Repair and Human Health, ed S. Vengrova. (Rijeka: INTECH Open Access Publisher), 177–238.
Velatooru, L. R., Baggu, C. B., and Janapala, V. R. (2016). Spatane diterpinoid from the brown algae, Stoechospermum marginatum induces apoptosis via ROS induced mitochondrial mediated caspase dependent pathway in murine B16F10 melanoma cells. Mol. Carcinog. 55, 2222–2235. doi: 10.1002/mc.22463
Wang, B., Waters, A. L., Valeriote, F. A., and Hamann, M. T. (2015). An efficient and cost-effective approach to kahalalide F N-terminal modifications using a nuisance algal bloom of Bryopsis pennata. Biochim. Biophys. Acta 1850, 1849–1854. doi: 10.1016/j.bbagen.2015.05.004
Wang, H., Chiu Lawrence, C. M., Ooi Vincent, E. C., and Ang Put, O. (2010). A potent antitumor polysaccharide from the edible brown seaweed Hydroclathrus clathratus. Bot. Mar. 53, 265–274. doi: 10.1515/BOT.2010.029
Wang, S., Wang, L.-J., Jiang, B., Wu, N., Li, X., Liu, S., et al. (2015). Anti-angiogenic properties of BDDPM, a bromophenol from marine red alga Rhodomela confervoides, with multi receptor tyrosine kinase inhibition effects. Int. J. Mol. Sci. 16, 13548–13560. doi: 10.3390/ijms160613548
Wang, X., Chen, Y., Wang, J., Liu, Z., and Zhao, S. (2014). Antitumor activity of a sulfated polysaccharide from Enteromorpha intestinalis targeted against hepatoma through mitochondrial pathway. Tumor Biol. 35, 1641–1647. doi: 10.1007/s13277-013-1226-9
Wang, X., and Zhang, Z. (2014). The antitumor activity of a red alga polysaccharide complexes carrying 5-fluorouracil. Int. J. Biol. Macromol. 69, 542–545. doi: 10.1016/j.ijbiomac.2014.06.017
Wichard, T. (2015). Exploring bacteria-induced growth and morphogenesis in the green macroalga order Ulvales (Chlorophyta). Front. Plant Sci. 6:86. doi: 10.3389/fpls.2015.00086
Williams, D. E., Sturgeon, C. M., Roberge, M., and Andersen, R. J. (2007). Nigricanosides A and B, antimitotic glycolipids isolated from the green alga Avrainvillea nigricans collected in dominica. J. Am. Chem. Soc. 129, 5822–5823. doi: 10.1021/ja0715187
Winter, C., Bouvier, T., Weinbauer, M. G., and Thingstad, T. F. (2010). Trade-offs between competition and defense specialists among unicellular planktonic organisms: the “killing the winner” hypothesis revisited. Microbiol. Mol. Biol. Rev. 74, 42–57. doi: 10.1128/MMBR.00034-09
Xie, P., Fujii, I., Zhao, J., Shinohara, M., and Matsukura, M. (2016). A novel polysaccharide derived from algae extract induces apoptosis and cell cycle arrest in human gastric carcinoma MKN45 cells via ROS/JNK signaling pathway. Int. J. Oncol. 49, 1561–1568. doi: 10.3892/ijo.2016.3658
Xin, Y., Yin, M., Zhao, L., Meng, F., and Luo, L. (2017). Recent progress on nanoparticle-based drug delivery systems for cancer therapy. Cancer Biol. Med. 14, 228. doi: 10.20892/j.issn.2095-3941.2017.0052
Yang, L., Wang, P., Wang, H., Li, Q., Teng, H., Liu, Z., et al. (2013). Fucoidan derived from Undaria pinnatifida induces apoptosis in human hepatocellular carcinoma SMMC-7721 cells via the ROS-mediated mitochondrial pathway. Mar. Drugs 11, 1961. doi: 10.3390/md11061961
Yu, Q., Yan, J., Wang, S., Ji, L., Ding, K., Vella, C., et al. (2012). Antiangiogenic effects of GFP08, an agaran-type polysaccharide isolated from Grateloupia filicina. Glycobiology 22, 1343–1352. doi: 10.1093/glycob/cws096
Zhang, C., Li, Y., Shi, X., and Kim, S.-K. (2010). Inhibition of the expression on MMP-2, 9 and morphological changes via human fibrosarcoma cell line by 6,6'-bieckol from marine alga Ecklonia cava. BMB Rep. 43, 62–68. doi: 10.5483/BMBRep.2010.43.1.062
Zhang, C., Yang, F., Zhang, X.-W., Wang, S.-C., Li, M.-H., Lin, L.-P., et al. (2006). Grateloupia longifolia polysaccharide inhibits angiogenesis by downregulating tissue factor expression in HMEC-1 endothelial cells. Br. J. Pharmacol. 148, 741–751. doi: 10.1038/sj.bjp.0706741
Zhang, L.-X., Cai, C.-E., Guo, T.-T., Gu, J.-W., Xu, H.-L., Zhou, Y., et al. (2011). Anti-cancer effects of polysaccharide and phycocyanin from Porphyra yezoensis. J. Mar. Sci. Technol. 19, 377–382.
Keywords: cancer, marine natural products, seaweeds, intracellular signaling pathways, biodiversity, marine chemical ecology, Sphaerococcus coronopifolius
Citation: Alves C, Silva J, Pinteus S, Gaspar H, Alpoim MC, Botana LM and Pedrosa R (2018) From Marine Origin to Therapeutics: The Antitumor Potential of Marine Algae-Derived Compounds. Front. Pharmacol. 9:777. doi: 10.3389/fphar.2018.00777
Received: 21 January 2018; Accepted: 26 June 2018;
Published: 06 August 2018.
Edited by:
Cheorl-Ho Kim, Sungkyunkwan University, South KoreaReviewed by:
Yung Hyun Choi, Dong-Eui University, South KoreaSubbaRao V. Madhunapantula, JSS Academy of Higher Education and Research, India
Copyright © 2018 Alves, Silva, Pinteus, Gaspar, Alpoim, Botana and Pedrosa. This is an open-access article distributed under the terms of the Creative Commons Attribution License (CC BY). The use, distribution or reproduction in other forums is permitted, provided the original author(s) and the copyright owner(s) are credited and that the original publication in this journal is cited, in accordance with accepted academic practice. No use, distribution or reproduction is permitted which does not comply with these terms.
*Correspondence: Rui Pedrosa, rui.pedrosa@ipleiria.pt